Self-Dosed Electromyography Biofeedback Via Wearable Electronics In Spinal Cord Injury: A Feasibility Study
Cotton, R. James1,2, Lee, KunHyuck2, Chung, Ha Uk2, Rogers, John2
1 Shirley Ryan AbilityLab (Chicago), 2Northwestern University
ABSTRACT
Electromyographic (EMG) biofeedback has been shown to enhance recovery of muscle control for subjects with incomplete spinal cord injury. However, adoption of this therapy is hindered by requiring expensive equipment and trained personnel. We aimed to overcome these limitations by developing an affordable, wearable sensor platform that can be employed by subjects to self-dose biofeedback therapy. We utilized recent advances in flexible and stretchable electronics to design an EMG sensor encapsulated in silicone with integrated electrodes interfacing to the skin via an adhesive hydrogel. This low-profile wearable sensor has wireless charging, Bluetooth connectivity, and also includes a 9-axis inertial measurement unit. The sensor continuously monitors activity throughout the day and records it to onboard memory. We also developed a suite of smartphone apps that supply biofeedback through games. Finally, we performed a feasibility study of this platform with a subject who had acute incomplete paraplegia over a period of 60 days during acute inpatient rehabilitation. He wore the sensor over his vastus medialis, which showed only trace activation on admission, and was able to control the games. The EMG activity acquired during biofeedback games showed both qualitative and quantitative improvements throughout admission. Now that the feasibility of self-dosed EMG biofeedback with wearable sensors has been validated, further work will be required to replicate the efficacy studies from laboratory studies of using this technology to improve motor recovery.
INTRODUCTION
Traumatic spinal cord injury is estimated to effect approximately 18,000 people per year in the US alone[1], and can result in a devastating loss of function, including profound weakness below the level of injury. Electromyographic (EMG) biofeedback has been shown to enhance recovery of muscle control for subjects with incomplete spinal cord injury [2]–[4]. However, adoption of this therapeutic modality is hindered by the need for expensive equipment and trained personnel. We aimed to overcome these limitations by developing an affordable, wearable sensor platform that can be employed by subjects to self-dose biofeedback therapy.
METHODS
Study Design
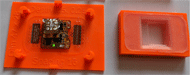
Platform design
Wearable Sensor
We utilized recent advances in flexible and stretchable electronics to design a comfortable wearable electromyography sensor (Figure 1). The design was fabricated using a 0.1mm thick flexible circuit board. The electrodes are mechanically decoupled from the main sensor board with serpentine wires, making it robust to twisting, stretching and rotation. They are then embedded through a bottom layer of silicone with the rest of the circuitry floating above it. Similarly, the battery is connected to the main board via serpentine wires allowing it to be positioned compactly in the final packaging. A Qi antenna is mounted below the main circuit module with accompanying electronics allowing wireless charging of the battery with industry-standard charging modules. It also provides a degree of stiffening to the main board protecting it from mechanical failures. The EMG signals are amplified and bandpass filtered before being digitized by the microprocessor, which also handles the Bluetooth connectivity. A 3D printed mold was used to cast a silicone shell that is completes the encapsulation.
Firmware on the sensors digitizes the EMG data at 2Khz and applies additional digital bandpass filtering. The waveform length algorithm [5] was used to extract EMG activity from subsequent 20 millisecond windows. The firmware exposes both the raw digitized EMG and the extracted EMG via corresponding Bluetooth characteristics. To optimize power consumption, the amplifiers are put into a low power mode when there is no subscriber to the characteristics. The firmware also allows continuous logging while the user is wearing the sensor by taking a sample of the EMG activity and writing this to microprocessor memory. With the data sampled every 5 seconds the sensor can store 4 hours of logging.
Smartphone Software
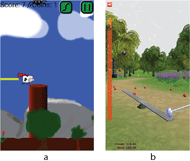
An accompanying software suite was developed for the Android platform. This includes a core service that handles the Bluetooth connectivity to fetch the EMG activity. It also periodically downloads the continuous logging data to prevent the sensor memory filling up. Two biofeedback games then connect to this Android service, allowing them to be controlled by the EMG data (Figure 2).
The first game “Flutter Cow” is an open source adaption of the popular game “Flappy Bird”. When the EMG activity crosses a programmable threshold, it simulates a click on the screen and makes the game character climb higher then resume drifting down. The player must reduce their EMG activity close to baseline to click again. The game character must be guided between various targets to progress.
The second game “PowerHammer” requires the player to generate sustained EMG activation over a two second window. Based on the strength of activation, this pulls back a hammer which then drives a ball upward. Rewards indicate to the user when they exceed their prior maximal activation.
Cloud Framework
Data from smartphone applications are transferred to Google Firestore. This platform has the advantage of transparently handling offline writing and then synchronization to the cloud when online. Uploaded data includes the continuous activity logs, EMG streams during gameplay, and gameplay performance and duration. Google Firestore also allows cloud computations to analyze the data such as tracking the amount of sensor wear time per day. The data can also be directly downloaded into python for further analysis. Finally, a web-based dashboard allows easily visualizing the activity of subjects using the sensors.
Participant
The subject in the feasibility study was a 30-year-old man with a traumatic T12 AIS C spinal cord injury. He was admitted to acute inpatient rehabilitation 22 days after his injury and enrolled in this study 5 days after admission. Surface EMG activity was recording from a sensor placed over his right vastus medialis. On enrollment he had trace activation of this muscle (with knee extension graded a 0 by Medical Research Council Muscle Scale several days prior on admission ASIA examination). At the end of the study this had improved to a 3 by MRC scale.
Procedure
Recruitment and informed consent occurred before the study began. After this, it was confirmed that volitional changes in surface EMG activity could reliably be obtained from the vastus medialis, which was the most clinically active muscle below his level of injury. The subject was then guided through how to apply and remove the wearable sensor, which included placing a hydrogel adhesive on the electrodes, which then adhere to the skin, and covering the sensor with an adhesive dressing.
A link to the app store was given to the subject who installed the biofeedback smartphone software on his device. He was then guided through how to connect to the sensor and play the biofeedback games. No specific instruction of amount of gameplay was provided to the subject, but rather he was instructed to play ad lib. He was requested to charge the sensor overnight on the wireless charging station when the battery was low and reapply it in the morning.
Analysis
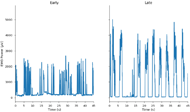
The times and durations of gameplay were retrieved from the cloud database as well as the corresponding EMG activity streams. The gameplay EMG activity was visualized to look for qualitative changes in activation patterns. As a proxy measure for maximal volitional EMG activity (MVEA), for each gameplay session the 99th percentile of EMG activity was measured (referred to as gameplay MVEA). The baseline activity was also computed from the 1st percentile. The weekly gameplay MVEA was computed by averaging the gameplay MVEA for each one-week window after enrollment.
RESULTS
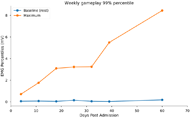
Figure 3 shows samples of EMG activity during gameplay from earlier (2 weeks) versus later (4 weeks) into the admission. Qualitatively it is apparent the subject is able to recruit greater and more sustained activation later into his admission. This partially reflects a transition from the “Flutter Cow” game to “Powerhammer”, which encourages more forceful and sustained activation, but the same trend was observed within games.
Figure 4 shows the weekly gameplay MVEA through the 60 days of monitoring. This shows a clear increase in the maximal activity throughout the admission. This trend was consistent is across a range of upper percentiles. This show that improvements in electromyographic activity can be monitored during recovery through the use of biofeedback games.
DISCUSSION
We successfully developed a platform achieving our aims in the form of a wearable electromyographic sensor with accompanying smartphone game allowing real-time biofeedback. They are flexible and stretchable and can be worn continuously throughout the day. They also are battery powered with wireless charging and data communication. Data from the platform, including EMG from both continuous monitoring and game play as well as game use is transparently synchronized to a secure cloud database. This novel overcomes many of the barriers to wider utilization of this EMG biofeedback, namely cost and difficulty of use (note this device does not have FDA approval for any use).
Additionally, this feasibility study demonstrated that self-dosed biofeedback with wearable EMG sensors and smartphone games is a viable therapeutic strategy for subjects with spinal cord injury. It demonstrated the realized sensors could be worn throughout the day and were sufficiently sensitive to allow control of biofeedback games despite only trace clinically detectable activity. The cloud infrastructure allowed monitoring activity and analyzing the data without requiring the further input from the subject. Recovery of muscle activation could be tracked with use by monitoring the maximal EMG activity during gameplay.
This improvement cannot be attributed to the biofeedback games, as the subject was undergoing physical and occupational therapy during this study, as well as the process of natural recovery. Future work will be needed to optimize the engagement of subjects with biofeedback games and to replicate the results from prior laboratory-based efficacy trials. Changes in EMG activity through this form of monitoring will also be correlated with changes in other functional outcomes like strength and walking performance.
[1] M. E. M. De Biase, F. Politti, E. T. Palomari, T. E. P. Barros-Filho, and O. P. De Camargo, “Increased EMG response following electromyographic biofeedback treatment of rectus femoris muscle after spinal cord injury,” Physiotherapy, vol. 97, no. 2, pp. 175–179, 2011.
[2] B. S. Brucker and N. V. Bulaeva, “Biofeedback effect on electromyography responses in patients with spinal cord injury,” Arch. Phys. Med. Rehabil., vol. 77, no. 2, pp. 133–137, 1996.
[3] K. Govil and M. M. Noohu, “Effect of EMG biofeedback training of gluteus maximus muscle on gait parameters in incomplete spinal cord injury,” NeuroRehabilitation, vol. 33, no. 1, pp. 147–152, 2013.
[4] D. Tkach, H. Huang, and T. A. Kuiken, “Study of stability of time-domain features for electromyographic pattern recognition,” J. Neuroeng. Rehabil., vol. 7, p. 21, 2010.