LIMITS AND USER SATISFACTION OF USING LOW-COST ROBOTS FOR MATH MEASUREMENT ACTIVITIES
Kim Adams1,2 and Al Cook1
1Faculty of Rehabilitation Medicine, University of Alberta, Canada, 2Glenrose Rehabilitation Hospital, Edmonton, Alberta, Canada
INTRODUCTION
Children who have severe physical limitations often cannot engage in manipulative activities like their able- bodied peers. As students, it can be difficult for them to be actively involved in learning activities, especially when "hands-on" activities are used to enhance learning (Eriksson, Welander, & Granlund, 2007). Assistive robots have been used to enable children with physical disabilities to manipulate items in educational activities, for example: science lab activities such as bringing items closer for sensory inspection (Howell & Hay, 1989; Howell, Martz, & Stanger, 1996), putting a glass over a burning candle to extinguish it (Kwee & Quaedackers, 1999) or mixing solutions, planting seeds, and plugging in electrical wires to make a radio (Eberhart, Osborne, & Rahman, 2000); art activities such as pasting items onto an art collage (Eberhart et al., 2000); or drawing lines to match questions and answers on a math worksheet (Smith & Topping, 1996). However, these were primarily brief trials with a few participants and the robots were not widely distributed. As Howell (2005) put it in his review of robotic devices as assistive and educational tools, the "lack of substantive, consistent exposure to a stable robotic device has led to interventions that are trials... too brief in duration and too few subjects to be able to achieve any level of generalizability" (p. 858).
One reason for not having consistent exposure to robots was the high price of early robotic systems. Some early researchers tried using inexpensive toy-like robots but they were criticized about "forcing cheap robots to barely meet [children's] needs rather than developing robotic systems that are truly well suited for educational purposes" (Lees & LePage, 1994, p. 298). Years later, Howell (2005) stated that it will take years to develop an easy-to-use, cost- effective, and reliable assistive robot for classroom use, and once developed, it will take more time to develop appropriate educational activities.
Recent studies utilized low cost Legoi Mindstorms robots for play activities and found that children with disabilities had similar positive gains while using Lego robots as in previous robot studies with more expensive robots (e.g., Cook, Adams, Volden, Harbottle, & Harbottle, 2010). Lego robots are robust compared to early toys, but they, too, have some limitations (e.g., cannot lift a heavy payload, veer off course over long distances, fine accuracy limitations). Based on experiences with Lego and other robots, Cook, Encarnação and Adams (2010) outlined desired robot design requirements for easy-to-use, cost- effective, and reliable assistive robots for classroom use. Despite their limitations Lego robots provide an opportunity to develop educational activities today, while more robust technology is being developed.
This study examined Lego robotic system use in math measurement activities and addressed the following questions:
- What are the key features and characteristics of a Lego robot that limit its effectiveness in math measurement activities?
- Do those limitations affect how well a user can demonstrate their understanding of math concepts and procedures using the robot?
- What is user satisfaction with using the robots for the math activities?
METHOD
Participants
A 12 year old girl, 10 year old boy and 14 year old girl with cerebral palsy participated in the study (Participants 1, 2 and 3). An Evaluation Team participated in interviews relating to robot limitations. The team consisted of a speech language pathologist, two special education teachers, two occupational therapists, and an adult who uses a speech generating device (SGD) and other means of augmentative and alternative communication.
Materials
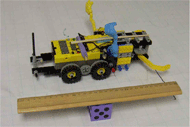
A Lego Mindstorms RCX robot with a gripper, moveable pen, and spindle to hold a spool of string was used for hands-on math measurement activities. Items to be manipulated were mounted on either the robot or a block (Figure 1). The participant could maneuver items on the robot by moving the robot forward, back, left or right. He/she could move items on the blocks, by grasping the block with the robot gripper, and then moving the robot.
All participants controlled the robot through their SGD. They all used a Vanguardii II SGD activated using two head- rest mounted switches, in step scanning. The infrared (IR) output of the SGD was used to control the robot. The SGD interface is described elsewhere (Adams, 2011b).
Methodology
The participants underwent a training protocol where they practiced the manipulation tasks that would be required in the subsequent math activities (Adams & Encarnação, 2011). Math activities were taught by a Special Education teacher. The length measurement lessons from Levels 1 and 2 of the Math Makes Sense resource guides were used (Pearson Education Canada, 2007, 2008). There were ten 30 to 90 minute sessions over 8 weeks. Participants compared, sorted, and ordered items in the first level. In the second level they measured items with non-standard units (e.g., straws) and then compared and ordered the items using the resulting length measurement.
Picture in picture videos were created with a view of the speech generating device SGD screen within a broad view of the participant and the activity. All session videos were observed by the investigator to annotate events when there seemed to be a problem using the robot during the activity. The events were coded using NVivoiii, qualitative analysis software which allows user defined coding of multiple types of documents (e.g., videos and text). Video segments demonstrating the problematic events were shown to the Evaluation Team to solicit opinions on how the problems might have affected how the participant could demonstrate their understanding of math concepts and procedures using the robot.
In each activity, the participants did the manipulation two ways: (1) by directly controlling the robot (robot), and (2) by observing the teacher do the manipulation and answering her questions regarding whether she was doing it correctly (observing and guiding teacher). After each activity participants were asked which method they preferred. They also completed a survey after the study regarding overall preference and ease of use.
RESULTS
The most frequent events where there were problems using the robot during the activity are presented here. Participants could not see if the ends of the item on the robot lined up with the comparison item when the items were far away (i.e., parallax: when objects seem to shift relative to one another depending on the angle of view). Compensation strategies used to address this issue were: the orientation of the table and/or items on the table were changed or the items were brought closer to the participant; a card or file folder was placed at the end of the comparison item (which bent over when the participant drove the robot into it); the teacher or investigator held their finger at the end of the item and also at the end of the comparison item (e.g., one finger on the spool at the back of the robot and the other finger on the start position for measuring).
The adapted items were sometimes difficult to manipulate, e.g., the straw units on the blocks sometimes bent so they did not line up exactly. To compensate for these issues the teacher or investigator straightened the straws as required or magnets were used on blocks or rods so they could snap together. The magnets were not used until the participant had already demonstrated that they knew the concept of lining up units tip to tip.
The robot steps were sometimes too big to stop exactly lined up with items or to place units tip to tip (due to sending a discrete IR command from the SGD). When the magnets were used, they compensated for this issue. Plus, if the participant attempted to move forward and backward to get closer to the baseline, the teacher interpreted this as an indication that they were trying to line it up and she placed the item in the exact location. This strategy was described by one of the Evaluation Team teachers as similar to when children with less severe disabilities attempt to do a task. If the teacher sees the child's intention and that he/she is close, the teacher will put the item where it is supposed to be (essentially behaving like the magnet).
The robot did not drive perfectly straight, especially when it had to go long distances (e.g., over 1 metre). To compensate, the teacher or investigator nudged the back of the robot straight while the participant drove the robot or the participants sometimes compensated by making a small turn.
There was one activity that was too difficult for any of the participants to accomplish with the robot - measuring a curved item.
Tables 1 and 2 show the participant's preference for way of doing the manipulation activities and their responses to the post-study satisfaction survey.
Activity Number | Observe and guide teacher | Do with robot |
---|---|---|
Activity 1 | Participant 1 Participant 2 Participant 3 | |
Activity 2 | Participant 1 Participant 2 Participant 3 | |
Activity 3 | Participant 1 Participant 2 Participant 3 | |
Activity 4* | Participant 2 | Participant 3 |
Activity 5* | Participant 2 | Participant 3 |
*Participant 1 did not do Activities 4 and 5. |
not at all | not really | sort of | a bit | a lot | |
---|---|---|---|---|---|
The robot was easy to use |
P2 P3* |
3* |
P1 P3* |
||
I liked using the robot to work on measurement |
|
P1 P2 P3 |
|||
I like using the robot more than telling my EA what to do |
P2 |
P1 |
P3 |
||
* Participant 3 broke the question into parts: she found some tasks "a lot", some "a bit" and some "sort of" easy. |
DISCUSSION
There were several robot issues that limited the accuracy with which participants could perform tasks, but the strategies used by the teacher and/or investigator compensated for the limitations. These compensation strategies were not deemed by the evaluation team as interfering with their interpretation of what the participant was demonstrating about their understanding of math concepts and procedures. The team felt that the compensation strategies merely augmented the robot and/or environment and allowed the participant to perform the tasks with the required accuracy.
There were other factors that contributed to the robot being effective, or not, in the math measurement activities such as the participant's skills and abilities, the appropriateness of the activities themselves, and the SGD interface. This paper examined only the robot and how it performed in the math activities. The other factors are covered elsewhere (Adams, 2011a). Measuring a curved item was an inappropriate activity to expect to accomplish with the robot - it was too difficult for all of the participants. Taking everything into account, the participants found the robot to be "sort of" easy to "a lot" easy use (Table 2).
In spite of the robot limitations, all of the participants preferred to use the robot instead of observing the teacher in the Level 1 activities (Table 1). In Level 2, Participants 1 and 3 continued to prefer to use the robot instead of observing the teacher; however, Participant 2 began to prefer observing the teacher over using the robot. The Level 2 activities required more accuracy, length of time and effort (e.g., one activity required the robot to travel long distances in order to measure how tall the participant was in straws). He indicated that he would have preferred to use the robot if the items to measure were shorter. Participant responses about preferred way to manipulate in the post- study survey (Table 2) are consistent with the preferences from Table 1.
Arthanat et al. (2007) state that "the user’s satisfaction with the [assistive technology] device is in essence derived on the basis of the effectiveness and efficiency of participation in activities". Robot effectiveness, from the participant's point of view would be how accurately they could accomplish the tasks using the robot as a tool. From a teacher's point of view, robot effectiveness would be how well the participant could demonstrate their understanding of the math concepts and procedures. The Evaluation Team also pointed out how the robot was an effective learning tool because the participants were actively engaged in the concepts being taught. Efficiency relates to the amount of time and effort to do the tasks. The participant's perception of effectiveness and efficiency did not negatively affect satisfaction for Participants 1 and 3. They were very satisfied using the robot regardless of their accuracy, length of time or effort. However, Participant 2's perceived effectiveness and efficiency did influence his satisfaction.
CONCLUSION
This study investigated the limitations of using of low- cost Lego robots in math measurement activities. It was expected that the Lego robots would have some inaccuracies, and limitations were observed during the activities. However, the robots had sufficient capability to accomplish these math measurement activities, an activity which inherently requires accuracy, and only required some minor compensation strategies by the teacher. These compensation strategies did not influence how a team of teachers, occupational therapists and speech language pathologists felt the participant was demonstrating his/her understanding of math concepts and procedures. In spite of the robot limitations, participants found the robots easy to use and were generally satisfied using the robots for the activities.
While we wait for an ideal robot to be developed, appropriate educational activities can be performed using low-cost Lego robots so that more children with disabilities can be actively engaged in "hands-on" school activities. Since not only researchers, but teachers, therapists and parents can easily replicate the Lego robots, it follows that children may engage in a greater variety of activities, on more occasions, for longer periods of time, thus maximizing the likelihood that assistive robots can have a positive, generalizable impact on children’s learning.
REFERENCES
Adams, K. (2011a). Access to math activities for children with disabilities by controlling lego robots via augmentative communication devices. University of Alberta, Edmonton, Alberta.
Adams, K. (2011b). Involving Users in the Design of a Speech Generating Device Interface for Lego Robot Control Paper presented at the Annual RESNA Conference, Toronto, ON.
Adams, K., & Encarnação, P. (2011). A Training Protocol for Controlling Lego Robots via Speech Generating Devices. Paper presented at the 11th European Conference for the Advancement of Assistive Technology, Maastricht, The Netherlands.
Arthanat, S., Bauer, S. M., Lenker, J. A., Nochajski, S. M., & Wu, Y. W. B. (2007). Conceptualization and measurement of assistive technology usability. Disability and Rehabilitation: Assistive Technology, 1- 14.
Cook, A., Adams, K., Volden, J., Harbottle, N., & Harbottle, C. (2010). Using Lego robots to estimate cognitive ability in children who have severe physical disabilities. Disability and Rehabilitation: Assistive Technology, 1-9.
Cook, A., Encarnação, P., & Adams, K. (2010). Robots: assistive technologies for play, learning and cognitive development. Technology and Disability, 22(3), 127- 145.
Eberhart, S. P., Osborne, J., & Rahman, T. (2000). Classroom Evaluation of the Arlyn Arm Robotic Workstation. Assistive Technology, 12, 132-143.
Eriksson, L., Welander, J., & Granlund, M. (2007). Participation in Everyday School Activities For Children With and Without Disabilities. Journal of Developmental and Physical Disabilities, 19, 485–502.
Howell, R. (2005). Robotic devices as assistive and educational tools for persons with disabilities. In D. Edyburn, K. Higgins & R. Boone (Eds.), Handbook of Special Education Technology Research and Practice
(pp. 849-862). Whitefish Bay, WI Knowledge by
Design.
Howell, R., & Hay, K. (1989). Software-Based Access and
Control of Robotic Manipulators for Severely Physically Disabled Students. Journal of Artificial Intelligence in Education, 1(1), 53-72.
Howell, R., Martz, S., & Stanger, C. A. (1996). Classroom applications of educational robots for inclusive teams of students with and without disabilities. Technology and Disability, 5, 139-150.
Kwee, H., & Quaedackers, J. (1999). POCUS Project: Adapting the Control of the MANUS Manipulator for Persons with Cerebral Palsy. Paper presented at the ICORR '99: International Conference on Rehabilitation Robotics, Stanford, California.
Lees, D., & LePage, P. (1994). Will robots ever replace attendants? Exploring the current capabilities and future potential of robots in education and rehabilitation. International Journal of Rehabilitation Research, 17, 285-304.
Pearson Education Canada. (2007). Math Makes Sense 1. Toronto, Ontario Pearson Education Canada.
Pearson Education Canada. (2008). Math Makes Sense 2. Toronto, Ontario Pearson Education Canada.
Smith, J., & Topping, M. (1996). The introduction of a robotic aid to drawing into a school for physically handicapped children: a case study. British Journal of Occupational Therapy 59(12), 565-569
ACKNOWLEDGEMENTS
We would like to thank members of the I Can Centre for Assistive Technology at the Glenrose Rehabilitation Hospital for their advice and assistance.