Morgan Redfield, Goeran Fiedler PhD, Brian Hafner PhD, Joan Sanders PhD
University of WashingtonAbstract
Persons with trans-tibial amputation are often challenged to regain a natural and efficient gait pattern with their prosthesis. Prosthetic fitting and rehabilitation therapy can be informed by gait analysis. Classical gait analysis commonly requires a well-equipped laboratory, which prohibits the long-term monitoring of subjects’ gait in a natural environment. Rehabilitation may be improved by the use of portable gait monitoring devices capable of measuring gait patterns over long time periods and without delay. This study investigated the validity of measurements taken by one such monitoring device. Gait data from a kinetic sensor mounted between the socket and prosthesis pylon was compared with data collected by a GAITRite electronic walkway. It was found that there is a strong correlation in stance and swing phase timing measures, which supports the intended use of the kinetic sensor for real-time gait monitoring in clinical rehabilitation.
Introduction
Rehabilitation after lower limb amputation has the general objectives of reconstituting a gait pattern that is stable, energy efficient, and that avoids un-physiologic loads and stresses of the remaining structure, e.g. the sound leg. Unimpaired gait is stable, efficient, and physiologic, and is therefore the standard by which rehabilitation efforts are measured. One feature of unimpaired gait is symmetry between legs. Many therapy regimens and research studies have adopted symmetry as an objective or outcome criterion [1, 2].
There is no uniform method for quantifying gait symmetry. Indices are commonly computed as the quotient of left-right difference and left-right average. One example is the Symmetry Index (SI) first introduced by Herzog [3]:
SI = (Xsound-X
prosthetic)/0.5*(X
sound+Xprosthetic) * 100% [equation 1]
Variable X may be any parameter that can be obtained by direct measurement. Parameters that have been reported in the literature include both spatial and temporal measures of kinematic and kinetic outcomes [4-7]. While such symmetry measures have been widely used in research studies, it must be noted that direct measurement of these outcomes in the clinic is often limited, due to availability of equipment and personnel trained to use it. More often, fitting of artificial limbs, as well as the administration of the subsequent gait training, is usually informed by subjective assessment methods rather than accurate measurements of gait symmetry.
Simplified methods of quantifying and optimizing walking symmetry that do not require the use of an instrumented gait analysis laboratory have therefore been proposed. Instrumented treadmills offer advantages, such as “the rapid collection and comparison of temporal and kinetic parameters of gait for multiple successive strides, at a constant known speed, without forcing subjects to target their footsteps” [8], and have been used to study asymmetry of outcomes in non-amputees [9-11]. Another alternative is wearable sensors, portable devices that can be on the body to measure parameters of interest. Wearable sensors offer the advantage of allowing data collection irrespective of the environment, and have recently been used to study gait asymmetries. Assessment of symmetry using wearable sensors can be based on lateral trunk accelerations [12] or bipedal ground contact pressure [13].
One challenge to measuring asymmetry is that most systems require that both limbs measured, which makes hardware extensive, obstructive, and susceptible to motion artifacts. The possibility to integrate sensor hardware into the structure of an artificial limb overcomes this limitation. However, since only variables for the prosthetic leg can be obtained with sensors integrated into a prosthesis, direct assessment of gait symmetry (in persons with unilateral amputation) is not possible. However, it may be possible to derive useful measures of temporal asymmetry from the timing of stance and swing phase of a single leg. Unilateral measurement of force through a prosthetic leg may also provide information that serves as a reliable predictor of gait symmetry. The degree to which unilateral kinetic outcomes reflect bilateral gait asymmetry has not been studied. This information is needed to determine if integrated prosthesis monitors could be used to characterize asymmetry or predict gait quality in persons with unilateral amputation.
Purpose
The purpose of this proof-of-concept study was to investigate the correlation of unilateral kinetics variables to bilateral gait symmetry.
Hypothesis
It was hypothesized that the optimal unilateral stance phase/swing phase ratio of 62/38 as determined by a prosthesis-mounted axial force sensor correlates with optimal left/right symmetry of 50/50 as determined by step length and step width, computed using a conventional gait analysis system.
Methods
To validate the use of an axial kinetic force sensor for out-of-clinic monitoring of prosthesis users, the stance and swing times found using a kinetic sensor (PCB Piezotronics, Depew, NY) were compared to stance and swing times found using a GAITRite Electronic Walkway (CIR Systems, Inc. Sparta, NJ).
Equipment
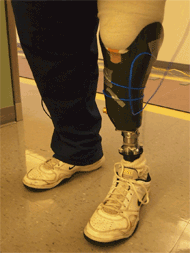
The GAITRite electronic walkway is an instrument for measuring the temporal and spatial parameters of a subject’s gait. The walkway is 61cm wide by 366cm long. As a subject ambulates over the walkway, the GAITRite system captures the geometry, timing, and relative arrangement of each footfall. These measurements can then be used to calculate such parameters as stance time and swing time for each step. The walkway was connected to a laptop, and video was recorded to be able to synchronize step measurements based on the timing and step count from the start of the respective trials.
The kinetic sensor is a 3-axis force sensor (model #260A02) that can monitor axial force and medial-lateral and anterior-posterior shear. It has a dynamic range of 4.45 kN, and can measure force in both positive and negative directions. The housing is 6.5cm tall, 5.5cm wide, and 5.5cm long, and has a mass of 510g. The top is a female pyramid adaptor and the bottom is a standard four bolt pattern.
A custom signal conditioning circuit was used to power the kinetic sensor and amplify the output signal. The amplified force signals were then sampled at 80Hz using a 10 bit A/D converter and saved on a Galaxy Nexus smartphone (Google, Mountain View, CA; Samsung, Seoul, South Korea) carried by the subject. An 80Hz sampling rate was deemed acceptable as most of the energy in gait is concentrated below the resulting Nyquist frequency of 40Hz [14, 15]. The signal conditioner and power circuitry were placed in a belt-pack to be worn by the subject. The belt-pack transmitted data over USB to the smartphone carried by the subject. A custom application on the smartphone then saved the force signals to a file for subsequent post-processing.
Experimental Protocol
The experimental protocol was approved by the University of Washington Institutional Review Board (IRB). A single subject was recruited from a local prosthetic clinic to pilot test the system. Inclusion criteria were trans-tibial amputation, use of an endoskeletal prostheses, and ability to walk comfortably and unaided for 30 minutes. Exclusion criteria were an age under 18, a weight over 125 kg, severe visual or auditory impairments, and use of a prosthesis that did not provide enough clearance to accommodate the kinetic sensor.
Upon providing informed written consent to participate in this study, the subject doffed his prosthesis. A prosthetist documented the original alignment of the prosthesis and installed the kinetic sensor between socket and prosthetic foot by replacing the original pylon (Figure 1). Alignment and length of the prosthesis were preserved during this process. The subject donned the prosthesis again, and walked for several minutes to assure that any differences in prosthesis weight and inertial characteristics caused by the additional hardware did not affect the gait pattern.
The smartphone application was then started and data collection begun using the kinetic sensor. The subject was asked to walk back and forth over a GAITRite mat at a self-selected walking speed. Data were collected for every pass using both the GAITRite mat and the kinetic sensor. Four experimental conditions were investigated (normal walking, purposeful limping, 4 lb. ankle weight on prosthesis, 4 lb. ankle weight on unaffected limb) in a random order. For the normal walking, weighted prosthesis, and weighted leg conditions, the subject was instructed to walk as normally as possible. For the purposefully limping condition, the subject was asked to favor their prosthetic leg as they walked, e.g. place more weight on their prosthesis. They were asked to walk to the end of the GAITRite walkway, turn around, and come back. They performed this procedure four times without stopping for each condition. At the conclusion of the tests, the kinetic sensor was removed and the prosthesis was reassembled to its initial state.
Analysis
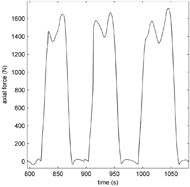
The data from the sensor was copied from the smartphone to a desktop computer and post-processed using MathWorks Matlab 7.12.0 (Natick, Massachusetts). The subject’s prosthetic leg swing and stance phases were determined by thresholding the axial force (Figure 2) signal. If the force exceeded the threshold, it was determined that the subject’s prosthetic leg was in stance phase. If the axial force did not exceed the threshold, it was determined that the subject’s prosthetic leg was in swing phase. The threshold used to discriminate stance and swing was determined by manually inspecting the force data.
The swing and stance phase durations were computed and a swing/stance ratio was calculated as swing duration divided by stance duration. The swing phase duration, stance phase duration, and ratio were compared to the values calculated by the GAITRite software. Bivariate correlation was computed in IBM SPSS Statistics for Windows (Version 19.0. Armonk, NY: IBM Corp.)
Data post processing included the computation of a symmetry index for each pass over the GAITRite walkway. Step length and step width were extracted from the GAITRite data and inserted in a version of [equation 1]. Resulting symmetry indices were averaged for each trial and plotted against the stance/swing ratios for visualization of the effect of gait symmetry changes on the variable of swing/stance ratio. No statistical evaluation was indicated for this single case proof-of-concept study.
Preliminary Results
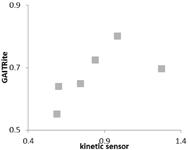
One subject participated in this study. The subject was a 50 year old male with a trans-tibial amputation of the left leg due to physical trauma. The subject’s weight was 85.3kg, height was 167.6 cm, and time since amputation was four years. The subject’s prosthesis was of endo-skeletal design and used pin and lock suspension.
The subject completed all trials within two hours, not reporting any significant fatigue. Walking trials were completed in the order: Normal walking, purposeful limping, walking with an additional weight on the prosthetic ankle, and walking with an additional weight on ankle of the contralateral leg.
Bivariate correlation analysis for steps concurrently measured by the GAITRite system and the pylon-integrated kinetic sensor (shown in Figure 3) resulted in a Pearson coefficient of R=0.631 (p=0.179). After removal of one outlier, identified by the unusual large temporal difference of the respective step cycle to the adjacent step cycle, and attributed to a measurement error, the coefficient increased to R=0.944 (p=0.016).
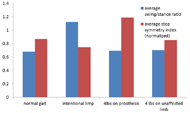
The collected data did not support the correlation of bilateral symmetry indices and unilateral swing phase/stance phase ratios as hypothesized. The relationship of these variables in the observed four conditions is illustrated in Figure 4.
Discussion
It was shown that a force sensor mounted in line with the pylon of a subject’s prosthesis is capable of making measurements that correlate well with the measurements made with a GAITRite electronic walkway. This may indicate that the force sensor can be used for gait monitoring of prosthesis users. Such a monitoring tool would be of use to clinicians and researchers in monitoring people outside of the laboratory or clinic.
Preliminary data support the assumption that variables that are extracted from the kinetic sensor are closely correlated to the same variables measured with the electronic walkway. However, there was no clear indication that the correlation between stance/swing ratio and measures of spatial gait symmetry was as strong as was hypothesized.
A pronounced step-by-step variability was observed in most all measurement variables (stance phase duration, swing phase duration, step width and step length) and appeared to be independent of the intervention conditions. This incidental finding was unexpected and may be characteristic of the individual tested. Variability in outcomes will have to be explored in future work with additional subjects. Other limitations of this study include the accuracy of synchronizing data from the electronic walkway and the integrated sensor by means of a video recording. An improved method for synchronizing data may mitigate possible error.
Future work will be directed at fully validating this method of gait monitoring in a larger sample to ensure its accuracy in gait data collection in the general population of persons with trans-tibial prostheses. This is a prerequisite for taking advantage of the integrated kinetic sensor’s mobility and smartphone connectivity in clinical applications such as gait training, optimization of prosthesis alignment, and activity monitoring.
Acknowledgements
We would like to thank Colin Dietrich and Kate Allyn for their help with the data collection.