Home Assessment Of Grasp Development In Infants
Hsin-Hung Kuo1, 2, Jing Wang1, 2, Manon Schladen2, 3, Michael Taylor1, Sahana Kukke4, Peter Lum1, 2
1The Catholic University of America, School of Engineering, Biomedical Engineering Department,
2Rehabilitation Engineering Research Center – D.C.
3Georgetown University Medical Center
4National Institutes of Health, Division of Neuroscience in National Institute of Neurological Disorders and Stroke
INTRODUCTION
Learning to use the hands to grasp or reach an object is an important milestone in neurological development during infancy. Hand movements that are initially spontaneous in nature are gradually tuned by sensory and motor experience and lead to functional grasping over the first year of life [1]. Delays in motor development can have sensory-motor consequences that range from minor difficulties to severe motor disabilities that prevent independent use of the hand [2]. Therefore, infants should be screened for different developmental pathways, motor delays, and/or motor disabilities, which are the most commonly detected problems among infants in the first years of life [3, 4]. However, it is questionable whether early developmental delay is indicative of later developmental disability [5]. Abnormalities or delays diagnosed early in life may be transient and eventually fade away as the central nervous system matures [6]. Nevertheless, infants who are reported to be initially delayed in the development of motor abilities are often referred for developmental therapy [7]. The therapeutic approach involves repetitive practice in an enclosed environment that is engaging for the infants, and is stronger if provided at home by means of an enriched environment, (i.e. a home organized to encourage the infant to perform specific tasks that are tailored to the developmental needs of the infant). The parent should be actively and positively engaged with the child to facilitate and promote learning. The home environment should also include safe toys, adequate for the infant's ability level, to pose learning challenges along with family interactions [8].
Sometimes, infants’ reaching and grasping (motor) development is delayed but not detected by parents/caregivers. The earlier a baby gets therapy for motor delay, the better the outcome. However, the standard spacing of healthy baby visits to the pediatrician makes it less likely that delay will be recognized and therapy started early. To overcome this obstacle, researchers have started to develop quantitative measurements of developmental milestones which aimed to quickly and efficiently identify infants at-risk for developmental delays [9]. We have developed a quantitative upper extremity sensory-motor assessment system for home use by parents and caregivers. We have built a play structure that can record high definition video of the infant’s upper extremity movements, and measure the power and precision of the grasp when the infant handles toys that give sensory (tactile, visual, auditory) feedback of grasp force. By quantifying grasp behaviors in response to tactile, visual, and audio biofeedback of the infant’s grasp force, we can build a developmental profile of sensory-motor integration. Finally, infants and their parents will be included in the design of the entire system to ensure successful home adoption. Creating objective methods to diagnose motor delays early and manipulating motor behaviors through biofeedback can increase the developmental potential of infants at risk for delay in motor development. In this paper, we describe our prototype system and report on data collected in the homes of volunteer participants.
METHODS
Participants and ethical considerations
We enrolled 8 infants (4 boys and 4 girls) undergoing typical development. The infants were tested at the age of 3 months. The inclusion criteria include: (1) full-term birth, i.e. gestational age greater than 37 weeks and (2) normal pregnancy and delivery. Participants were excluded for congenital orthopedic and significant visual or hearing deficits. Ethics approval was obtained from the Institutional Review Board of the Catholic University of America. Written informed consent was obtained from the parent prior to administration of the test to each infant participant.
Settings: Hand use and grasp sensor (HUGS) System
The approach in this study was to use the HUGS to measure grasp force and arm movements in typically developing infants. The project staff traveled to the homes of participants and showed parents how to set up and operate the HUGS system (Fig.1).They were given detailed, printed instructions on how to ready the system, position the infant relative to the camera and instrumented toys, and initiate and collect video and force data. Our goal was to measure the gripping behaviors of the baby. In this analysis, we processed the signals gathered from force sensors in conjunction with the video and calculated a number of metrics related to grasping performance. The HUGS also includes use of a RGB-D camera capable of 3-D tracking of arm movements. This aspect of the data will not be presented here.
Circuit design and making the toy
Electronic control unit
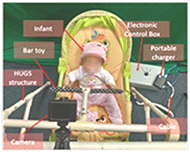
To power and record the sensors and deliver feedback, we used an Arduino R3 microcontroller. This Arduino also has an SD card option to store data. The controller and associated electronics were all placed in the control box with a switch on top to start the data collection and change feedback modes. The switch on the control box has four options: vibration mode, light mode, sound mode, and close (turn off) (Fig.2). The data for each trial is stored in the SD card flash memory. The system is powered by a 5 volt rechargeable Li-ion battery.
Toy design and Sensors
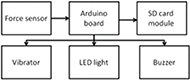
Toy effect feedback
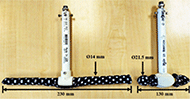
Our research purpose was to observe the infant’s response to controlled sensory stimulation in familiar environmental conditions (at home). The toys in the HUGS system were developed by integrating force sensors, vibration feedback, visual feedback, and auditory stimulations into one toy. When a baby grasped the toy, it would provide sensory feedback in proportion to the force of the grasp. Furthermore, the feedback of vibration, visual and auditory stimuli upon successful contact with the toys served also to direct the infant’s attention to the toy and increase toy-infant interaction.
Force calculation
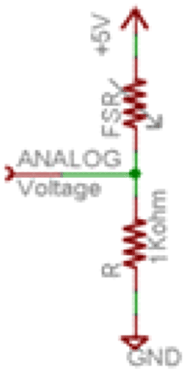
The FSR was wired to the Arduino analog input according to the integration guide available from the vendor [10]. The voltage between the fixed pulldown resistor and the variable FSR resistor is connected to the analog input of the Arduino (Fig. 4). We can adjust the range of measurement of gripping force by changing the value of the pulldown resistor. The bar toy has one long FSR sensor embedded into each side. The sensor was calibrated by applied known weights to several toy locations. System calibration data showed some differences in the grasping force when measured across different locations and orientations of the toys. However, these differences in grasping force can be corrected through the video analysis process where the grasping locations and grasp orientations are identified/inferred from observations.
Data analysis
In the quantitative study, force sensor data were extracted for each infant session. Data were analyzed by calculating their peak force, mean force, force standard deviation and the number of times the baby gripped the toy. The IBM SPSS statistics 25 software was used for statistical analysis. In order to test if parents could collect data independently, we used paired sample t test and intra-class correlation coefficient (ICC) to compare the data collected with our guidance and the data collected by the parents themselves. The significance level of paired sample t test was p<0.05 and the ICC values were interpreted as follows: less than 0.4 is indicative of poor reliability, values between 0.40 and 0.59 indicate moderate reliability, values between 0.60 and 0.74 indicate good reliability, and values greater than 0.75 indicate excellent reliability [11].
Grip characteristics | Bar toy | Candy toy | ||
---|---|---|---|---|
paired sample t test | ICC Coefficient | paired sample t test | ICC Coefficient | |
RH grasp frequency | 0.676 | 0.526 | 0.119 | 0.592 |
RH grasp duration | 0.662 | 0.954 | 0.691 | 0.626 |
Right hand mean force | 0.464 | 0.920 | 0.099 | 0.211 |
Right hand peak force | 0.499 | 0.883 | 0.076 | 0.646 |
LH grasp frequency | 0.256 | 0.456 | 0.408 | 0.204 |
LH grasp duration | 0.339 | 0.294 | 0.847 | 0.523 |
LH mean force | 0.900 | 0.188 | 0.538 | 0.406 |
LH peak force | 0.713 | 0.862 | 0.408 | 0.338 |
RESULTS
Grip characteristics |
Toy Type |
Gender |
Right hand force (g) |
Left hand Force(g) |
---|---|---|---|---|
Peak force of infants with one hand on the toy | Bar toy | Female | 999 | 452 |
Male | 509 | 1024 | ||
Candy toy | Female | 571 | 201 | |
Male | 302 | 306 | ||
Peak force of infants with both hands on the toy | Bar toy | Female | 603 | 480 |
Male | 490 | 458 | ||
Candy toy | Female | 105 | 68 | |
Male | 598 | 538 | ||
Mean force of infants with one hand on the toy | Bar toy | Female | 82±66 | 53±19 |
Male | 106±51 | 109±56 | ||
Candy toy | Female | 52±30 | 46±15 | |
Male | 68±49 | 56±19 | ||
Mean force of infants with both hands on the toy | Bar toy | Female | 130±117 | 63±41 |
Male | 89±36 | 96±71 | ||
Candy toy | Female | 42±3 | 35±8 | |
Male | 78±56 | 47±29 |
The test took place at the infants’ home. During the first visit, we guided the parent operating the HUGS system and let the baby play with the bar toy and candy toy in different modes for 2 minutes each. The parents were asked to collect a session of data on their own within one week of the first visit. Infant play was spontaneous during each task, both for the choice of hands to use in unimanual or bimanual grasping actions and for the choice of the type of grasp and force exerted. Parents were instructed to guide the infant to grasp the toy if the infant was not actively playing with the toy spontaneously. During the study, we found that some three-month-old infants needed assistance from parents for the exploration of toys and we also found that when some infants’ palms are in a closed fist, they needed parents to guide their grasp of the toy. After several times of parents’ guidance, most infants can play with the HUGS toys actively by themselves.
We examined the force sensor data and the synchronized video and manually identified the instances of toy grasp, eliminating instances when the parent’s hand was on the toy or the infant’s arm was resting on the toy without a true grasp. We calculated metrics of mean force, peak force, grasp duration and number of grasps. The results of statistical analysis showed no differences between the two collection sessions on any of the metrics (paired t-tests, p>0.05). For the bar toy, right-hand grasp duration, right-hand mean force and right-hand peak force were reliable when comparing data collected by the parents with and without our guidance (ICC > 0.75, see results in Table 1).
Hand | Toy type | Gender | Accumulated grasp frequency (number of times) |
Accumulated duration of grip (second) | ||
Right hand | Left hand | Right hand | Left hand | |||
One hand |
Bar toy | Female | 69 | 36 | 562.7 | 248.5 |
Male | 48 | 61 | 177.3 | 603.4 | ||
Candy toy | Female | 37 | 32 | 176.7 | 115.9 | |
Male | 62 | 52 | 241.5 | 181.7 | ||
Both hands | Bar toy | Female | 13 | 56 | ||
Male | 33 | 156.1 | ||||
Candy toy |
Female | 4 | 6.9 | |||
Male | 18 | 52.3 |
In terms of the overall average strength, the mean grip force of the infants was around 100g (Table 2). Because of the different shape and size of the bar toy and the candy toy, the bar toy has a larger grasp force range than the candy toy. When infants used one hand to grasp the toys, their peak force and mean force on the bar toy were higher than that on the candy toy. We also noticed some differences between the male infants and the female infants: when with one hand grasped the bar toy, female infants’ right hand peak force and grip duration was much higher than the left hand, while male infants showed the reverse trend. With both hands on the bar toy, female infants had higher peak force and mean force with their right hand while male infants showed more balanced forces between their both hands during bimanual grasp. For frequency of grasp and hand grip duration analysis (Table 3), we found that when three-month-old infants use one hand they grasped the toy longer and more frequently than when they used both hands. Since every infant subject was given 6 minutes to play with each toy, from the accumulated grip duration data in Table 3, we found that the accumulated grip time was around 1700 seconds for the bar toy and 800 seconds for the candy toy, which represents about 60% and 28% of the overall play time with each toy. It shows a good level of acceptance for the HUGS system.
DISCUSSION
The present research was designed to investigate, in infants at three months old, unimanual and bimanual grasping performance with toys designed for cylindrical grasp and spherical grasp. Preliminary testing has revealed high acceptance of the toys. The infants were very interested in our designed smart toys and therefore executed the expected type of play. All these aspects make the device useful for monitoring infants’ grasping movements. This study confirms the potential usefulness of the device as a tool for continuous monitoring and quantitative measuring of infant hand function and motor development. Moreover, our system can potentially be used with infants at high risk for developmental motor delays in order to evaluate any potential differences from the healthy infants.
The sensory feedback from the toys can potentially be a useful intervention. Previous research has shown that augmented feedback enhances motor learning and it can be used effectively in rehabilitation. Augmented feedback is defined as information that cannot be elaborated without an external source. It is provided by technical displays, such as visual, hearing, and haptic modalities [12]. By this definition, our toys provide augmented feedback using tactile, visual, and auditory modalities. Future work will be to collect longitudinal data from healthy infants and infants at risk of developmental delay between 3 and 9 months of age. These data, collected by the parents independently, will be used to establish grasp development profiles than can potentially be used to assist diagnosis of infants who can benefit from an early intervention.
REFERENCES
- Bhat A, Galloway J. Toy-oriented changes during early arm movements: Hand kinematics. Infant Behavior and Development. 2006;29(3):358-372.
- Cecchi F, Serio SM, Perego P, Mattoli V, Damiani F, Laschi C, et al., editors. A mechatronic toy for measuring infants’ grasping development. 2008 2nd IEEE RAS & EMBS International Conference on Biomedical Robotics and Biomechatronics; 2008: IEEE.
- Van Haastert I, De Vries L, Helders P, Jongmans M. Early gross motor development of preterm infants according to the Alberta Infant Motor Scale. The Journal of pediatrics. 2006;149 (5):617-622.
- Jeng S-F, Yau K-IT, Chen L-C, Hsiao S-F. Alberta infant motor scale: reliability and validity when used on preterm infants in Taiwan. Physical therapy. 2000;80(2):168-178.
- Marlow N. Neurocognitive outcome after very preterm birth. Archives of Disease in Childhood-Fetal and Neonatal Edition. 2004;89(3):F224-F228.
- Bartlett DJ. Comparison of 15-month motor and 18-month neurological outcomes of term infants with and without motor delays at 10-months-of-age. Physical & occupational therapy in pediatrics. 2000;19 (3-4):61-72.
- Cecchi F, Serio S, Del Maestro M, Laschi C, Sgandurra G, Cioni G, et al., editors. Design and development of “biomechatronic gym” for early detection of neurological disorders in infants. 2010 Annual International Conference of the IEEE Engineering in Medicine and Biology; 2010: IEEE.
- Lucca K, Horton R, Sommerville JA. Keep trying!: Parental language predicts infants’ persistence. Cognition. 2019;193:104025.
- Ho ES, Torres W, Prosser L, Johnson MJ, editors. Ailu: An Affordable Sensorized Toy for Detection of Neuro and Motor Delays in Infants. 2019 IEEE 16th International Conference on Rehabilitation Robotics (ICORR); 2019: IEEE.
- FSR Force Sensing Resistor Integration Guide and Evauation Parts Catalog. 2010. Interlink Electronics.
- Cicchetti DV. Guidelines, criteria, and rules of thumb for evaluating normed and standardized assessment instruments in psychology. Psychological assessment. 1994;6(4):284.
- Bertucco M, Sanger TD. Current and emerging strategies for treatment of childhood dystonia. Journal of Hand Therapy. 2015;28(2):185-194.