Jing Wang1,2, Peter S. Lum1,2, Hsinhung Kuo1,2, Thanh Phan1,2, Sang Wook Lee1,2
1Catholic University of America, School of Engineering, Department of Biomedical Engineering,
2Rehabilitation Engineering Research Center (RERC–DC)INTRODUCTION
Upper extremity paresis of stroke survivors has disabling effects on all facets of life. In particular, their behavioral maladaptation, “learned non-use” of the more affected limb, may initiate a vicious cycle in which non-use and poor performance reinforce each other, further exacerbating their functional impairments [1].
Different rehabilitation practices have been implemented for upper extremity training for stroke patients, but their efficacy in overcoming learned non-use of the affected limb is limited. Conventional clinician-guided repetitive practice of upper extremity movements can improve outcomes for stroke survivors, but access to the clinic for therapy is often costly and limited by geography or lack of transportation [2], limiting their overall effectiveness in promoting the use of more impaired arm. Constraint-Induced Movement Therapy (CIMT) forces the use of the paretic limb by constraining the movements of the non-paretic limb for most waking hours [3-5], which could eventually promote the use of paretic limbs. However, multiple studies have questioned applicability of the CIMT techniques, considering them too demanding, not suitable for bimanual functional tasks or trainings, and difficult to implement for severely impaired patients [6-8]. Ballester et al. developed the Reinforcement-Induced Motor Therapy (RIMT), to be used in combination with CIMT for overcoming learned non-use and motor impairments [9]. The results showed a significant increase in arm-use during training sessions and these patients continued to exhibit further significant gains in the UE Fugl-Meyer at a 12-weeks follow-up. However, the current RIMT training scenarios all limited hand movements to be performed over a surface providing anti-gravity support, and most scenarios still constrained the use of the less affected limb.
We recently developed a virtual-reality (VR) training environment to promote the voluntary use of the more-impaired limb. We altered visual feedback to “virtually” enhance/augment the motor function of the more impaired limb by amplifying the movement distance of the paretic arm in a VR environment. This method is designed to lower the metabolic energy requirements associated with movements of the paretic limb and to influence the patient’s perceived performance, thereby promoting their use.
The aim of this study is to systematically evaluate the efficacy of the developed VR training environment among healthy population in unsupported reaching tasks, as a preliminary step to assess the potential of this training mechanism for the stroke population. Specifically, we examined 1) to what extent the visual amplification of hand movements can change the performance of the rewarded limb; 2) whether the adjusted limb performance can alter people’s hand use choices in unsupported reaching tasks. We examined the correlation between the kinematics of participants’ movements and their responsiveness to the reward and the visual amplification of hand movements.
METHODS
Settings
The altered visual feedback in the VR system, Visual Amplification of Hand Movements, was programmed in Unity. When no amplification is implemented, the avatar’s hand positions in VR are based on the subject’s hand positions in the real world. When a level of amplification implemented, for example 50% amplification to the right hand, the subject’s right avatar’s hand position in VR was calculated as the distance between the right hand position in reality to its starting position multiplied by 1.5 in x, y, z axes. As a result, when subjects reached for the same target, with visual amplification on, the right limb doesn’t need to move as high/far as the left side (Fig. 1c).
Participants
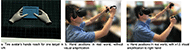
Thirty-one right-handed participants (mean age 21.9 ± 2.9 yrs; 15 females), naive to the experiment, participated in this study. All participants were recruited from the Catholic University of America through posted flyers in the campus and word of mouth, according to the following inclusion criteria: over the age of 18 years old, neurologically healthy, and strongly right-handed according to at least 80% score on the Edinburgh Handedness Inventory [2]. People were excluded from this study if they had any conditions that restrict their arm movements or limit their capability of using a Head Mounted Display (HMD) for a VR environment. The study was approved by the Catholic University of America Institutional Review Board and the written informed consent was obtained from all participants before participating in the experiment.
Experimental Procedure
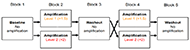
We used a randomized cross-over design for this study (Fig. 2). Each subject completed 5 experimental blocks of 100 unsupported reaching movements towards different targets in an immersive VR system (Oculus Rift; Facebook Technologies, LLC.). No trunk restriction was used in the experiments and subjects were instructed to move at their comfortable speed. Two levels of virtual amplification of the movements of the right hand, x1.5 amplification and x2 amplification, were implemented in the 2nd and 4th blocks. The sequence of two amplifications levels was counter balanced across subjects.
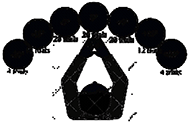
In each reaching task trial, subjects can use either hand to reach to a cube target (12cm×12cm×12cm) that appeared at one of the 14 locations in VR, with two height levels (eye/shoulder level) at each of the 7 horizontal angles (0°; ±7°; ±15°; ±30°), centered with respect to subject’s midsagittal plane (Fig. 3). All target locations were were scaled to 80% of each subject’s arm length, within the subject’s comfortable range of motion. Participants could reach all targets comfortably with either hand. Within each block, the target appeared at the ±30° locations on 8 trials, the ±15° on 24 trials, the ±7° locations on 40 trials, and at the center location on 28 trials (Fig. 3). This distribution is chosen to increase the sampling rate at locations in which participants were expected to use both hands (ambiguous locations). The eccentric, 30°locations, are included to decrease the likelihood that subjects would adopt a strategy of using only one hand to reach to all targets. The sequence of target locations was randomized. Each subject was given at least 5 mins break between two experimental blocks. In each reaching trial, the subject was required to put his/her hands on his/her laps as a starting position. After the start position was achieved (automatically detected by the system), a cube target will show up in the VR environment and the subject can use either hand to reach the target. Once an avatar’s hand, either the right hand or the left hand, reached a target, the target will disappear, and this ended the trial. The preset time intervals between reaching trials was 3 seconds if the subjects completed the last reaching trial and the start position for the next trial is achieved. If the subjects took more than 3 seconds to complete the two criteria above, the system would wait to start the next trial until the two completion criteria were achieved. There was a short beep at the timepoint when a target showed up in the VR environment to draw subjects’ attention. Subjects took about 1.5 hour to complete all steps of this experimental procedure of this study.
We also accessed subjects’ awareness about the altered visual feedback in the VR environment. We included a debriefing survey at the end of the testing to assess whether the subject was aware of the visual amplification of hand movements in experimental blocks. Subjects were asked if they had noticed any changes in the VR system over the course of the experiment; if the task got easier, harder, or stayed the same for the right hand and left hand; if they used one hand more than the other, and if this pattern changed over the course of the experiment.
Data analysis
The hand choice that subjects used to reach each target, target location, and timestamps in these experimental blocks were collected in the logging system. Subjects’ both hand controller positions and headset positions were recorded at 90 Hz and stored on a computer. Our main outcome measure is the percentage of right-hand use, to measure subjects’ hand selections in the study. We calculated the total right-hand use percentage across all targets for each block. This value was also calculated for each target. This procedure was performed separately for each block.
Two statistical tests were conducted to test our two assumptions: 1) there was no significant differences of right hand use percentage among the three baseline blocks (1st, the 3rd, and the 5th blocks) without any visual amplification implemented; 2) the order effect (whether participants got the 50% amplification level first or the 100% amplification level first) on the right hand use percentage was negligible. Two-way mixed ANOVA with the amplification level as the within-subjects factor and the order type as the between-subjects factor were used to test the two assumptions.
To examine the effect of visual amplification of hand movements on hand selection patterns in reaching tasks, we calculated the right-hand use percentage (RHP) of each block, and used two-sided paired T-test to compare the RHP of the block with visual amplification to the prior block without visual amplification implemented for both levels of visual amplification. Correlation tests (Pearson, Spearman, Chi-square) were done to examine the relationship between subjects’ responsiveness (RHP) and subjects’ awareness. Significance level was set to 0.05 for all conducted statistical tests. Descriptive statistics are reported as mean ± SE.
RESULTS
The visual amplification of the hand movements significantly increased the use of the right hand (RHP; p=0.002), while there was no significant difference (p=0.752) in the RHP among the three blocks without visual amplification of hand movements (block 1: 65.4%± 14.5%; block 3: 64.6±18.7%; block 5: 63.3%±17.7%). The between-subjects factor order type (p=0.199) and the interaction between order type and amplification level (p=0.584) were not significant, which supported our two assumptions.
When the RHP of the block with visual amplification (either x1.5 or x2) was compared to the prior block without visual amplification for all 31 participants, the RHP significantly increased for both x1.5 visual amplification (73.3%±17.3% vs. 66.2%±16.8%, p=0.003), and the x2 amplification (72.9%±17.7% vs. 63.5%±17.3%, p=0.013) compared to the prior block without visual amplification. No significant difference was found between the RHP of two different amplification levels.
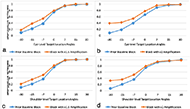
Figure 4 show the target location based right hand use percentage in different experimental blocks. The usage of the right hand increased and the workspace of the right hand expanded to the left side of the center line when the visual amplification was implemented in the second block and the forth block, compared to the baseline and washout blocks without visual amplifications. We also analyzed the left boundary of the right-hand workspace during each experimental block. We found that when reaching targets at the seven eye-level locations (in 0°, ±7°, ±15°, and ±30° horizontal angles), when under the x1.5 amplification, the left boundary of the right-hand workspace significantly expanded to -15.4°± 10.7°, compared to -11.4°± 9.9° in the prior baseline block (p=0.028). When under the x2 amplification, the left boundary also significantly expanded (-20.1°± 11.8° vs. -12.7°± 9.4°, p<0.001). For the shoulder-level targets, the left boundary of the right-hand workspace significantly expanded to -18.6°± 11.6° with the x2 amplification (p=0.011), compared to -12.9°± 8.3° before.
No significant correlations were found between subjects’ awareness of the visual amplification and their right hand use percentage change. A significant correlation was observed between whether the participants noticed the changes in the experiment and their gender (Chi-Square test, p=0.002). Among the 17 participants who were aware of the changes in experimental blocks, 13 of them were male.
DISCUSSION
The visual amplification did show significant effect in altering subjects’ hand-use choices in unsupported reaching tasks, where the use percentage of their right hand significantly increased, and the workspace of this hand significantly expanded to the left side compared to the baseline blocks.
Our system was designed to be an implicit, reinforcement manipulation, altering the person’s internal sense of success and behavior through intrinsic processes, to promote the use of the rewarded limb and induce long-term changes in real-world behaviors. Surprisingly, around half of the subjects noticed the change in the system introduced by the visual amplification of hand movements and male subjects seemed to be much more sensitive and aware of this visual perturbation. However, we didn’t find significant correlations between subjects’ awareness of the reward and their performance responsiveness to the reward.
The novelty of this study includes that we augmented the upper extremity performance of the dominant side in 3D space while preserving the intended movement directions of subjects, and we examined hand choice in functional tasks. These results will be valuable to provide preliminary support and practical guidance when applying this treatment approach for upper extremity training of stroke patients. The limitations of this study include that the testing protocol was not designed to systematically test the retention of hand-selection choice shifting and we did not assess the right-hand use patterns in the real-world tasks after experiencing the reward in the VR system. Future studies are needed to investigate the effects of visual amplification of hand movements in chronic stroke population, and to examine the persistence effect on limb selection patterns in both the immersive VR environment and real-world settings.
REFERENCES
- Wolf, Steven L., Deborah E. Lecraw, Lisa A. Barton, and Brigitte B. Jann. "Forced use of hemiplegic upper extremities to reverse the effect of learned nonuse among chronic stroke and head-injured patients." Experimental neurology 104, no. 2 (1989): 125-132.
- Kwakkel, Gert. "Impact of intensity of practice after stroke: issues for consideration." Disability and rehabilitation 28, no. 13-14 (2006): 823-830.
- Taub, Edward, and Gitendra Uswatte. "Constraint-induced movement therapy: bridging from the primate laboratory to the stroke rehabilitation laboratory." Journal of Rehabilitation Medicine-Supplements 41 (2003): 34-40.
- Meinzer, Marcus, Thomas Elbert, Daniela Djundja, Edward Taub, and Brigitte Rockstroh. "Extending the constraint-induced movement therapy (CIMT) approach to cognitive functions: Constraint-induced aphasia therapy (CIAT) of chronic aphasia." NeuroRehabilitation 22, no. 4 (2007): 311-318.
- Corbetta, D., V. Sirtori, P. L. Moja, and R. Gatti. "Constraint-induced movement therapy in stroke patients: systematic review and meta-analysis." (2010): 537-544.
- Viana, Ricardo, and Robert Teasell. "Barriers to the implementation of constraint-induced movement therapy into practice." Topics in stroke rehabilitation 19, no. 2 (2012): 104-114.
- Page, Stephen J., Peter Levine, Sueann Sisto, Quin Bond, and Mark V. Johnston. "Stroke patients' and therapists' opinions of constraint-induced movement therapy." Clinical rehabilitation 16, no. 1 (2002): 55-60.
- Charlotte Brunner, Iris, Jan Sture Skouen, and Liv Inger Strand. "Recovery of upper extremity motor function post stroke with regard to eligibility for constraint-induced movement therapy." Topics in stroke rehabilitation 18, no. 3 (2011): 248-257.
- Ballester, Belén Rubio, Martina Maier, Rosa María San Segundo Mozo, Victoria Castañeda, Armin Duff, and Paul FMJ Verschure. "Counteracting learned non-use in chronic stroke patients with reinforcement-induced movement therapy." Journal of neuroengineering and rehabilitation 13, no. 1 (2016): 74.