Allonstride: An Exoskeleton For Stroke Patients' Rehabilitation
Cecilia Lou, Josh Ching
University of Ottawa, Biomedical Mechanical EngineeringProblem Statement/Research Question and Background
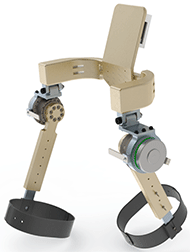
uOttawa Bionics is a multidisciplinary engineering team that aims to develop mechatronic medical devices to improve patients' quality of life and provide aid in rehabilitation processes. The team is currently working on an exoskeleton that can be used as a rehabilitation device to assist patients suffering from lower extremity motor disabilities caused by stroke. Pictured in Figure 1, the present prototype, called the AllonStride, is a 4-DOF hip-mounted exoskeleton that produces torque and relieves the load on leg muscles when walking. This torque that is produced by a motor gradually decreases as until the patient has fully recovered.
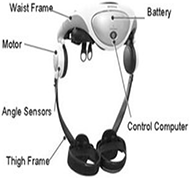
In order to build the AllonStride, extensive research was done on existing exoskeletons. Two were examined in particular. The Honda Stride Management Assist and the PH-EXOS developed by Honda Motor Company and Southeast University in Nanjing China respectively.
The Honda Stride Management Assist, pictured in Figure 3, is an exoskeleton that is available in Japan for the public to purchase [1]. It was designed to reduce the amount of body weight carried by the user's legs [1]. This system was developed to reduce strain and fatigue when walking, climbing stairs, and crouching [1]. The device uses two brushless DC motors and a rechargeable lithium battery. At the hip joint, it can provide up to 6Nm of flexor torque.
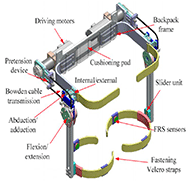
The PH-EXOS is an exoskeleton that was developed to assist patients with walking impediments by providing lower limb motion to simulate natural walking [2]. As can be seen in Figure 4, this 6DOF exoskeleton used AC servomotors with Bowden cables for transmission [2]. FRS sensors were also added in the thigh cuffs to collect experimental data on muscle contraction.[2]
Methods/Approach/Solutions Considered
When first designing the AllonStride, many options were considered. While many adjustments were made, there were four major designs that were altered. At first, hydraulics were considered as a method of actuation. However, further analysis determined that the pumps required to provide the desired power would result in an unreasonable increase in weight. As a result, a simpler and lighter alternative, electric actuation, was selected. Secondly, the original sensor system included a footswitch to determine the toe-off phase of the user's gait cycle. However, since the final designs of the exoskeleton did not extend to the user's foot, this idea was dropped. Instead, a series of three accelerometer-gyroscope packs were used. Thirdly, a Kalman filter was to be used as a control and as a gait analysis system. This had the ability to reduce noise and integration-related drag. Ultimately, a machine learning algorithm that compares the measured limb positions and accelerations with data collected from individuals with a healthy gait, were used to appropriately control the motor. Finally, in the manufacturing process, while multiple parts were initially planned to be 3D-printed, this was deemed inadequate when experimentally tested. For instance, aluminum was determined to be a superior option for the hinge pieces that allow for passive abduction and adduction of the leg due to the high friction that this part undergoes.
Description of Final Approach and Design
As mentioned, the AllonStride is a hip-mounted lower extremity exoskeleton. It is designed to sit on the patient's hips and has two braces that secure the patient's thighs. It is a powered exoskeleton and is designed to have four degrees of freedom (DOF).
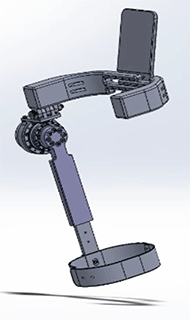
In the current prototype, a CHF-25-160-2UH Harmonic Drive interfaces with an EC90-Flat Brushless Maxon Motor (with the addition of an HD set screw adapter) at its input and a thigh piece fixed to the user's leg above the knee, is directly attached at its output (see Figure 4). As such, only the flexion and extension motions are actuated. The design allows abduction and adduction; however, this motion is not actuated.
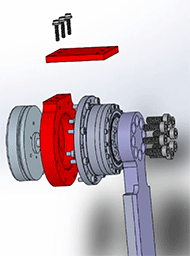
The red component in Figure 5 that holds a Harmonic Drive component. The thigh pieces were manufactured out of a high strength polymer (Ultem), this ensures the material can handle the wear and tear caused by the use of the suit.
To ensure that the suit fits the average person, the leg length, as well as waist and leg widths, are adjustable. The range of the thigh length is set to be adjustable between 43 and 73 cm while the hip breadth between 22.5 and 51.8. These values were used according to anthropometric data obtained from literature in [3]. Hip breadth is varied by adjusting the selected screw holes on the back piece and the fingers on the piece that are secured to the waist.
Two sensor packs consisting of a 3D accelerometer and a gyroscope are placed along the thighs to determine the orientation of the limbs and a 3D accelerometer is placed on the lower back to identify heel contact events using the methods described by Mansfield and Lyons in [4]. A Raspberry Pi reads the data acquired from the sensor packs and sends the information via WiFi to a desktop computer for analysis. This control system will utilize a machine learning algorithm that accurately compares the user's limb movement and acceleration and compares the date to that of a healthy gait; the device can then adjust and monitor movement to a higher degree of accuracy.
Outcome (Results of any outcomes testing and/or user feedback)
To validate the design, consultations were conducted under approval from the University of Ottawa's Office of Research Ethics and Integrity (File Number H-12-18-1382). The consultations took two forms: an online survey and in-person interviews. Using community contacts, face-to-face interviews were arranged with individuals with mobility impairments (stroke, injury, spinal injuries, etc.) as well as their caretakers (nurses, family members, doctors, physiotherapists, etc.). All potential participants (both caretakers and individuals with mobility impairments) were given the option to either do an in-person interview or participate in an online survey, based on their availability or personal preference.
The interviewed individuals had never previously used the proposed device. The interviews focus on their experiences with mobility impairment, rehabilitation and exoskeletons. The online survey was composed of very similar questions to the in-person interviews, without the option of physically interacting with the device. Instead, they were asked to provide feedback from images and videos.
At the time of submission, 2 individuals with mobility impairments, 4 caretakers were interviewed (2 family members, a physiotherapist and a medical doctor). General impressions were positive. One woman who had experienced a stroke was excited by the prospect of ascending stairs, a motion she has struggled with since her accident. To gain more physiotherapy in the months following her stroke, she mentioned she would sit at the door of the rehabilitation gym in the hospital in case another patient cancelled their appointment. Exoskeletons would help patients receive more care without the need for such extreme practices. One caretaker discussed the financial pressure caused by the cost of rehabilitation technology as an important barrier to many families, justifying the low-cost of the proposed device. None of the participants had any experience working with exoskeletons, indicating a lack of access to this promising technology.
The provided feedback will also be used to guide the design of future iterations of the device. For example, many participants expressed concern with regard to the bulkiness of the 3D printed hip pieces. More consultations will be carried out in the following months.
Cost (Cost to produce and expected pricing)
The total cost for the device is found in Table 1. The total cost to manufacture the device is $ 6,249 CAD, or $ 4,683 USD. This includes expensive manufacturing costs from one-off purchases; if mass-produced, the unit price would drop drastically.
Item | Cost |
---|---|
Padded suspenders and belt (Canadian Tire) | $120 |
Arduino Nano, general electric equipment and USB cables (Amazon) | $128 |
Wire, domain and website, gyroscopes, linear potentiometers and accelerometers (Variety of sellers) | $101 |
Raspberry Pi and Arduinos (Amazon) | $89 |
Harmonic Drive, Maxon controller, Maxon motor and HAL sensor | $4780 |
Wireless Module and SD cards (Amazon) | $66 |
Aluminum for motor support, hinges, and leg pieces | $138 |
Manufacturing motor support components | $622 |
3D Printed Components (printed at local Makerspace, Nylon & Ultem 1010) | FREE |
Screws and other fasteners | $205 |
Total cost | $6249 |
Significance
Every year 15 million people fall victim to strokes worldwide [5]. Of the 10 million who survive, approximately half are left with permanent disabilities [5]. Stroke is one of the leading causes of disability worldwide [6] and in the United States it is the leading cause for paralysis [7]. To reduce such motor impairments, rehabilitation is the most effective solution [6]. While conventional therapy relies on a therapist's skills and endurance, exoskeletons permit patients to heal/rehabilitate more independently for longer periods of time and with more intensity. [8] In addition, exoskeletons can collect patient data and produce precise repetitive movement. [9] Given the large/great number of people who suffer from temporary and permanent stroke-induced physical disabilities, there is a great demand for rehabilitation equipment to aid stroke patients. In an ageing population, there is a growing need for technology to assist in mobility. uOttawa Bionics' device answers this need.
References
- "Honda Walk Assist Mobility Technology," Honda. [Online]. Available: https://www.honda.com/mobility/walking-assist-technology. [Accessed: 11-Feb-2020].
- Q. Wu, X. Wang, F. Du, and X. Zhang, "Design and Control of a Powered Hip Exoskeleton for Walking Assistance," International Journal of Advanced Robotic Systems, vol. 12, no. 3, p. 18, Mar. 2015.
- [3] NASA, "ANTHROPOMETRY AND BIOMECHANICS," National Aeronautics and Space Administration, 7 May 2008. [Online]. Available: https://msis.jsc.nasa.gov/sections/section03.htm. [Accessed 8 August 2018].
- A. Mansfield and G. M. Lyons, "The use of accelerometry to detect heel contact events for use as a sensor in FES assisted walking," Medical Engineering & Physics, vol. 25, pp. 879-885, 2003.
- "The Internet Stroke Center," The Internet Stroke Center. An independent web resource for information about stroke care and research. [Online]. Available:http://www.strokecenter.org/patients/about-stroke/stroke-statistics/. [Accessed: 09-Feb-2020].
- S. M. Hatem, G. Saussez, M. D. Faille, V. Prist, X. Zhang, D. Dispa, and Y. Bleyenheuft, "Rehabilitation of Motor Function after Stroke: A Multiple Systematic Review Focused on Techniques to Stimulate Upper Extremity Recovery," Frontiers in Human Neuroscience, vol. 10, Sep. 2016.
- B. S. Armour, E. A. Courtney-Long, M. H. Fox, H. Fredine, and A. Cahill, "Prevalence and Causes of Paralysis—United States, 2013," American Journal of Public Health, vol. 106, no. 10, pp. 1855–1857, Oct. 2016.
- A. Cerasa, L. Pignolo, V. Gramigna, S. Serra, G. Olivadese, F. Rocca, P. Perrotta, G. Dolce, A. Quattrone, and P. Tonin, "Exoskeleton-Robot Assisted Therapy in Stroke Patients: A Lesion Mapping Study," Frontiers in Neuroinformatics, vol. 12, Jul. 2018.
- [9] D. Shi, W. Zhang, W. Zhang, and X. Ding, "A Review on Lower Limb Rehabilitation Exoskeleton Robots," Chinese Journal of Mechanical Engineering, vol. 32, no. 1, Aug. 2019.