Ashleigh Fults, Stephen Sundarrao, Stephanie Carey PhD
University of South Florida, Center for Assistive, Rehabilitation and Robotics Technologies
INTRODUCTION
For individuals with spinal cord injuries (SCI) who utilize manual wheelchairs for mobility, it is necessary to transfer into and out of their wheelchair frequently during the day. Transfers are needed to complete many daily activities including, but not limited to: using the toilet, entering a vehicle, and using a recreational wheelchair for a sport. Wheelchair users can be assisted in transfers, but independent transfers, wherein an individual transfers himself or herself unaided using only their upper limbs and torso promote rehabilitation and improved mental health. [1] Even with these possible benefits, safety remains paramount; an incorrect transfer can easily result in injury from a minor skin abrasion to a serious fall. Furthermore, even if a single transfer does not result in a fall or injury, repeated improper movements may cause pain, lead to musculoskeletal pathologies, or exacerbate preexisting damage to the upper limbs or shoulders. [2] Therefore, merely preventing a fall is not a sufficient goal for a transfer. A transfer technique which mitigates small injuries over time and successfully moves the individual to and from the target is necessary. In order to develop an optimized transfer technique and then train individuals in the technique, the current transfer technique must first be examined. One particularly beneficial activity for individuals with SCI are adaptive sports which allow the individual to compete in a sport while utilizing a manual wheelchair. Adaptive sports are especially important for this population as they provide competitors with socialization and personal fulfillment. [3] Specialized recreational wheelchairs are needed for such sports. One such specialized wheelchair is the hand cycle which is used in racing and differs in many aspects from the typical manual wheelchair. One key difference is the hand cycle's seat height. The purpose of this study was to compare the elbow kinematics of an independent wheelchair transfer from a manual wheelchair into a hand cycle at a seat elevation equal to that of the manual wheelchair and at a seat elevation of 10 centimeters below.
METHODS
Instrumentation
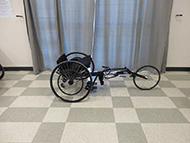
For this study, kinematics were collected using an eight-camera passive motion capture system, Vicon Nexus with Nexus 1.8.2 software. The hand cycle used was the Top End Eliminator Racing Wheelchair with Open V Cage (racer) (Figure 1).
Subject
This study was approved by the University of South Florida Institutional Review Board (IRB). To be included in this study, the subject had to be 18 years of age or older, a long term manual wheelchair user of two or more years, able to independently transfer without assistance, and have a low level SCI at T1 or below. Additionally, the subject was an active member of a local wheelchair sports team and utilized an unspecified hand cycle for racing, though they had not used the racer selected for this study prior to this participating in this study.
Procedure
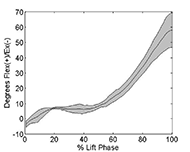
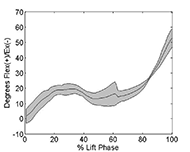
After the subject read, understood, and signed an informed consent form, they completed a short questionnaire and were weighed to ensure that they met the inclusion criteria and did not exceed the weight limit of the racer, respectively. To capture the subject's movements, twenty-five passive, reflective markers were adhered to the subject's upper limbs. The seat of the racer was adjusted to be at the same height as that of the subject's own wheelchair. Before the racer was secured in place, the subject was permitted to arrange and angle both the racer and their own chair as they would in an everyday situation. For the subject's safety, mats were placed around both wheelchairs, and research staff members were near to assist in the event of a fall. The subject then transferred unaided into the racer a total of three times. The subject was instructed to transfer at their own pace as they were in an ordinary situation. No instruction or direction for how to transfer was given to the subject outside of what they would normally do to transfer. The subject was given ample opportunity to rest between transfers. Next, the racer seat was lowered 10 centimeter, and again the subject arranged and angled the wheelchairs to their own preference. Once the same safety measures were in place, the subject transferred three times into the racer. All comments and observations made by the subject were recorded.
Analysis
From the literature, a transfer is broken into three phases. The phase of interest for this study is the lift phase, since it is the weight-bearing phase of transfer. [4] The start and end of the lift phase was defined as the point at which the subject's pelvis moved off the seat and the point at which the subject's pelvis was placed on the seat of the racer. Joint angles were calculated with the biomechanics analysis software, C-Motion Visual 3D, and the joint coordinate system used for the elbow was that recommended by the Standardization and Terminology Committee of the International Society of Biomechanics. [5] The mean and standard deviation of elbow angle was calculated for each arm from the three trials. The data was normalized to the percent of the lift phase.
RESULTS
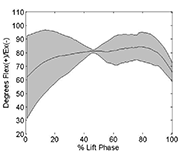
For the elbow angles reported, 0 degrees is considered the neutral position with the elbow in neither flexion nor extension. Elbow flexion is reported as positive degrees from the neutral position, and elbow extension is reported as negative degrees from the neutral position. For Figures 2-5, the mean elbow angle is denoted by a solid black line and one standard deviation above and below the mean is denoted by a gray region. The leading arm is defined as the arm which was placed on the racer during the lift phase of transfer, and the trailing arm is defined as the arm which remains on the subject's wheelchair during the lift phase of transfer. [6]
The mean elbow angle of the leading arm is a fairly smooth curve and a small standard deviation when the racer is set at the lower height (Figure 2). The mean elbow angle of the leading arm when the racer is at an equal height also has a fairly small standard deviation, but shows a spike at around 60% of the lift phase (Figure 3).
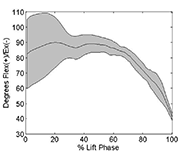
The mean elbow angle of the trailing arm when the racer is set to the lower height shows a large standard deviation throughout the lift phase except for around 50% of the lift phase (Figure 4. The mean elbow angle of the trailing arm when the racer is at an equal height shows a large standard deviation for only the first 50% of the lift phase (Figure 5). The large standard deviations for the trailing arms may be due to the subject attempting different movement strategies wherein the trailing arm is positioned at a different place on their personal chair. The subject may rely more on the leading arm to guide their body to the desired position on the racer, whereas the trailing arm is used more for propulsion and support.
DISCUSSION AND CONCLUSIONS
A similar study by Koontz et. al. shows similarly large deviations from the mean of the elbow angle during the lift phase for the trailing arm, but greater deviations from the mean of the elbow angle for the leading arm. [4] However, the comparison may not be sound due the greater number of subjects in the other study.
This study carried many limitations. A single subject many not show as much variation between techniques as multiple subjects may. This may contribute to the discrepancy between the standard deviations of the elbow angle of the leading arm in this study and the Koontz study. Furthermore, the subject was accustomed to transferring independently so much that they reported that they could complete a floor to chair and chair to floor transfer unaided. Because of the subject's proficiency and prior experience with recreational wheelchairs, they may have been much more at ease transferring which may have affected the results.
Continuing this study with more subjects would improve the results. Currently, more subjects are being recruited to further the study. Additionally, examining other upper limb joint angles and trunk tilt during transfer may better characterize the transfer technique in its entirety. Though not addressed in this publication, this study is also recording the muscle activation of the biceps brachii through electromyography (EMG) in order to support the kinematic findings. Notwithstanding these limitations, this case study is a good initial step to characterizing the current independent transfer technique, adds to the current body of knowledge of transfer kinematics, and sheds light on how to improve future studies.
REFERENCES
- Gagnon, D., et. al. (2003). Biomechanical analysis of a posterior transfer maneuver on a level surface in individuals with high and low-level spinal cord injuries. Clinical Biomechanics. 18, 319-31.
- Finley, M. A., et. al. (2005). Scapular kinematics during transfers in manual wheelchair users with and without shoulder impingement. Clinical Biomechanics. 20(1) . 41-8.
- Evseev, S. (2018) Adaptive physical education and adaptive sports within the mental health-care system, International Journal of Culture and Mental Health, 11(1), 109-112.
- Koontz, A. M., et. al. (2011). Development of custom measurement system for biomechanical evaluation of independent wheelchair transfers. Journal of Rehabilitation Research & Development, 48, 1015-28.
- Wu, G., et. al. (2005) ISB recommendation on definitions of joint coordinate systems of various joints for the reporting of human joint motion – Part II: shoulder, elbow, wrist and hand. Journal of Biomechanics, 38, 981-992.
- Gagnon, et. al. (2008). Trunk and upper extremity kinematics during sitting pivot transfers performed by individuals with spinal cord injury. Clinical Biomechanics. 23(3) pp. 279-90.
ACKNOWLEDGEMENTS
This study was partially funded by the Florida Department of Education, Rehabilitation Engineering and Technology Program.