Jorge L. Candiotti1,2, Sivashankar Sivakanthan1,3, Rory A. Cooper1,2,3
1Human Engineering Research Laboratories, VA Pittsburgh Healthcare System, 2Department of Bioengineering, School of Engineering, University of Pittsburgh, 3School of Health and Rehabilitation Sciences, University of Pittsburgh
INTRODUCTION
Tips and falls are a frequent consequence of wheelchair-related accidents in community settings [1]. There are 3.6 million wheelchair users in the US [2] in which 500,000 people use electric power wheelchair (EPWs) [3]. However, existing EPWs are limited primarily to use indoors [4] and outdoors over ADA compliant surfaces [5]. Studies have shown that EPW users face mobility difficulties when executing community-related activities due to exposure to challenging terrains. For instance, driving over differences in ground levels, potholes[6], drop curbs [7], and uneven surfaces may cause loss of stability which put users at risk of getting injured and damage their EPWs [8]. As result of these accidents, more than 100,000 wheelchair-related injuries are treated in emergency departments in the US every year, in which approximately 65-80% of these were due to tips and falls [9, 10]. Experienced EPW users often use the tilt-in-space seating function to adjust their center of mass to prevent any tips when driving up and down slopes [7]. However, this requires manual adjustments. Also, the lack of side tilt adjustment in EPWs put users at risk of tipping when driving on uneven surfaces [11]. In an interview with EPW users, interviewees highlighted the need for wheelchairs that could self-adjust or assist in overcoming these obstacles [12]. Few commercial EPW offer automated seat stability; however these are limited for seat adjustment in the median plane or their seating capabilities may not be adequate for people with complex mobility impairments [13, 14].
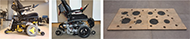
The paper proposes two alternative EPW dynamic suspensions to address these issues. These dynamic suspensions were the design iterations incorporated to the mobility enhancement robot (MEBot) EPW (Figure 1). MEBot consists of a Group 3 power seating system, footprint similar to EPWs, and a base frame with six height-adjustable wheels controlled by the dynamic suspensions [15]. The dynamic suspensions provided a larger vertical range of motion than common EPW suspension to automatically adjust its seat orientation with respect to the terrain. The first dynamic suspension consisted of pneumatic actuators (MEBot-pa) that adjusted the air pressure difference within their valves to control each wheel's height and overall damping system. A previous study demonstrated its stability on uneven terrains; but also, participants reported discomfort due to arbitrary wheel adjustments and delay to adjust the wheels' height [16]. In response, the second dynamic suspension was developed which consisted of electro-hydraulic actuators and spring in series (MEBot-ehas) to provide active and passive suspension, respectively. The goal of this study was to compare the driving performance and user's perception of safety and discomfort when using MEBot dynamic suspensions compared to common EPW suspensions on uneven surfaces.
Methods
A convenience sample of 10 participants were recruited for the study. The inclusion criteria included participants who were 18 years old or older, weigh less than 113.4 kg, able to tolerate sitting for two hours, had at least 1 year of experience using an EPW in indoor and outdoor environments, and used an EPW as their primary means of mobility (>8hours per day). Participants with pressure sores or back, pelvic or thigh pain were excluded from the study. The study was approved by the Veterans Affairs Pittsburgh Healthcare System Institutional Review Board. Participants were asked to complete a general questionnaire about demographics and information about their current EPW, wheelchair accidents, and commonly encountered driving environments. After completing the questionnaire, participants were randomized in the order for the use of three EPW suspensions (MEBot-ehas, MEBot-pa, and own EPW). If either MEBot was selected, their driving parameters and seating system were configured to meet participant's own EPW's characteristics. MEBot used a commercial EPW controller with a joystick that participants were familiar with. Participants received a video demonstration and driving training of MEBot. In addition, participants practiced driving with each MEBot suspension in the targeted driving task. The driving practice allowed participants to get used to the MEBot wheelchair to reduce selection bias towards their own EPW. After training, participants were asked to complete five trials with each EPW suspension in an engineered driving task. The driving task consisted of a series of potholes of 0.50 cm depth, up to 31 cm diameter in a 2.40-meter path (Figure 1). The task was based on a standardized wheelchair skills training [17] simulating damaged or uneven surfaces. A clinical investigator and two spotters followed each participant throughout the driving task to bring the EPWs to an immediate halt if a risk to the participant was perceived. To ensure participants' safety and controlled variables, the speed of the wheelchair was maintained at a maximum reasonable rate of 2.5 m/s.
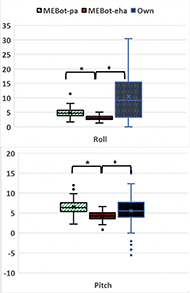
At the end of the study, participants were asked to complete a 5-point Likert scale questionnaire in safety, ease of use, and comfort for each EPW suspension. In addition, quantitative driving metrics were collected to measure the safety and driving performance of the three EPW suspensions. These metrics included absolute peak seat angles (pitch and roll), number of completed trials (no assistance, assistance, incomplete), and average completion time (seconds). The demographics questionnaire and outcome measures results were reported using descriptive analysis (e.g., means, standard deviation, percentages) and graphical techniques (e.g., box-plots, margin errors). Differences in driving metrics and participants' perception towards each EPW suspensions were analyzed using 1-way repeated measures Analysis of Variance (ANOVA). The level of significance was set α = 0.05 for all comparisons.
Results
Ten EPW users (5 male, 5 female) with an average age of 53.9 ± 11.47 years old and EPW driving experience of 21.2 ± 16.3 years were recruited for the study. Six participants used a mid-wheel EPW, 3 front-wheel and 1 rear-wheel drive EPW. All participants used a joystick as primary control method and reported to have at least one power seating function (i.e., tilt-in-space). The MEBot training time was approximately 15 minutes.
Participants completed all trials with the MEBot-ehas, 1 did not complete the pothole task with the MEBot-pa due to unnecessary movements of the device and discomfort, and 3 participants did not complete the potholes with their own EPW for different reasons: 1) fear of wheelchair breakdown, 2) dead batteries, 3) unsafe by spotters (Table 1). All participants required some level of physical assistance to complete each trial with MEBot-ehas (2/50 trials), MEBot-pa (28/50), and their own EPW (25/35). The average EPW speed was 0.27 m/s.
The MEBot-ehas suspension showed significant less changes in its seat angle back/forth (pitch direction) when traversing potholes compared to MEBot-pa (p=0.006) and participants' own EPW suspension (p<0.001). In addition, the MEBot-ehas suspension showed significant less changes in its seat angle sideways (roll direction) compared to MEBot-pa (p<0.001) and their own EPW suspension (p=0.003) as shown in Figure 2.
No statistically significant results were reported in user's perception of comfort (p = .104), safety (p=0.064) between EPW suspensions (Table I). On the other hand, participants reported higher comfort and safety responses when using MEBot-ehas compared to MEBot-pa suspension and their own EPW suspension. In terms of ease to use, participants reported that MEBot-ehas was significantly easier to use than MEBot-pa (p=0.009) and as similar to use as their own EPW suspension (p=0.519).
MEBot-ehas | MEBot-pa | Own EPW | ||
Completed trials (total = 50) |
No assistance | 96% | 34% | 20% |
With assistance | 4% | 56% | 50% | |
Incomplete | 0% | 10% | 30% | |
User's perception questionnaire 0 - Not satisfied 5 - Completed satisfied |
Comfort | 4.5 ± 0.5 | 2.6 ± 1.0 | 4.0 ± 1.3 |
Safety | 4.8 ± 0.4 | 3.5 ± 1.6 | 4.0 ± 1.2 | |
Ease of use | 5.0 ± 0.0 | 3.6 ± 1.4 | 4.4 ± 0.8 | |
Seat Angle Peaks (degrees) |
Pitch | 3.1 ± 0.7 | 4.8 ± 1.6 | 10.5 ± 7.6 |
Roll | 4.3 ± 1.1 | 6.5 ± 1.9 | 5.6 ± 4.1 |
Discussion
The MEBot-ehas suspension showed a better performance than its alternative MEBot-pa suspension and EPW suspension to reduce seat angle changes when driving on an uneven surface. In addition, participants complete all trials with MEBot-ehas with minimum physical assistance compared to the other EPW suspensions. The use of electro-hydraulic actuators with spring in series offered a smoother response rate and less unwanted movements compared to its previous iteration. This design improvement was also complemented with participants' perception of comforts, safety, and ease of use of MEBot-ehas compared to MEBot-pa suspension. While the user's perception results were not statistically significant between all EPW suspensions; participants rated high scores for MEBot-ehas and their own EPW when traversing on potholes. Despite of receiving no more than 15 minutes of training, participants felt comfortable and safe to use MEBot due to its similarities in driving and seating configurations as their own EPW. While participants were able to complete all trials with their own EPW; 50% of them required physical assistance when getting stuck. Common causes included the caster wheels getting stuck or limited traction to drive over the potholes. These results justified the response of six participants at the beginning of the study who reported to encounter potholes more than 3 times per week; but half avoid them if possible. As one participant pointed out that he/she wouldn't drive the EPW without spotters or a caregiver. Also, out of the three participants who did not attempt the driving tasks; one was afraid of driving on these surfaces due to a previous wheelchair accident in similar surfaces and another participant was aware of possible damage to his/her brand-new EPW. Likewise, participants were satisfied with the benefits of the MEBot-ehas to overcome these uneven surfaces while keeping them safe and comfortable. In is worth noting the discrepancy in participants' perception of safety compared to high changes in EPW seat angles when driving on potholes. While participant may feel safe; their own EPW reaches high seat angles that may put users at risk of tipping. The high seat angles in the pitch directions can be justified by the use of the tilt-in-space mechanism to shift their center of mass and create more stability or better grip in their drive wheels to drive on these surfaces. Note that the starting pitch angle in participants' own EPW was 8.3 ± 6.1° to tilt its seat. On the other hand, this seating configuration can be detrimental when facing up slopes. This position also limits user's visuospatial awareness of the environment; therefore, increasing the risk of getting injured and/or damaging their EPW in the process. It is worth noting that qualitative results should be complemented with quantitative metrics as much as possible. The use of an inertial measurement unit (IMU) was beneficial to quantify the safety of EPW. In addition, the sensor can be used to quantify the comfort as demonstrated in a previous study in similar environment conditions [18].
Study Limitations
The lack of significant differences could have been influenced by several factors. First, selection bias towards their own EPWs. This was minimized by accommodating testing EPWs to their own and getting familiar with environment. Second, while questionnaire results showed similar results in eha-MEBot and own EPW; they show no safety difference between EPWs. A larger sample size could increase the effect size to find significant differences. Third, a ceiling effect in the qualitative tools were observed. This was complemented with quantitative metrics which resulted in a discrepancy with participants' perception. Last, the test was performed in a controlled environment. The results of this study served as evidence of safety to evaluate the optimized MEBot dynamic suspension in outdoor environments for future studies.
Conclusions
The study evaluated the stability analysis of EPW dynamic suspension and passive suspensions in terms of driving performance and user's perception. The results demonstrated that the proposed EPW dynamic suspensions offered more stability than common EPW suspensions; hence reducing the risk of tipping and increasing EPW user's safety and comfort to drive on uneven surfaces. The study also demonstrated the design improvements of a EPW dynamic suspension using quantitative and qualitative metrics. The significance of these implications in important to improve the design of EPW technology to enhance user's confidence to navigate over these surfaces.
REFERENCES
[1] L. A. Rice, C. Ousley, and J. J. Sosnoff, "A systematic review of risk factors associated with accidental falls, outcome measures and interventions to manage fall risk in non-ambulatory adults," Disability and rehabilitation, vol. 37, no. 19, pp. 1697-1705, 2015.
[2] (2012). Americans with Disabilities: 2010 Household Economic Studies. [Online] Available: http://www.census.gov
[3] M. P. LaPlante and H. S. Kaye, "Demographics and trends in wheeled mobility equipment use and accessibility in the community," Assistive Technology®, vol. 22, no. 1, pp. 3-17, 2010.
[4] B. E. Dicianno and E. Tovey, "Power mobility device provision: understanding Medicare guidelines and advocating for clients," Archives of physical medicine and rehabilitation, vol. 88, no. 6, pp. 807-816, 2007.
[5] D. o. J. United States, 2010 ADA standards for accessible design. [Washington, D.C.]: Dept. of Justice (in English), 2010.
[6] S. Evans, C. Neophytou, L. de Souza, and A. O. Frank, "Young people's experiences using electric powered indoor - outdoor wheelchairs (EPIOCs): potential for enhancing users' development?," Disabil Rehabil, vol. 29, no. 16, pp. 1281-94, Aug 30 2007, doi: 10.1080/09638280600964406.
[7] C. Henje, G. Stenberg, J. Lundälv, and A. Carlsson, "Obstacles and risks in the traffic environment for users of powered wheelchairs in Sweden," Accident Analysis & Prevention, vol. 159, p. 106259, 2021.
[8] Henderson GV, Boninger ML, Dicianno BE, and Worobey LA, "Type and frequency of wheelchair repairs and resulting adverse consequences among veteran wheelchair users," Disability and Rehabilitation: Assistive Technology, pp. 1-7, 2020.
[9] H. Xiang, A. Chany, and G. A. Smith, "Wheelchair related injuries treated in USA emergency departments," Injury prevention, vol. 12, no. 1, pp. 8-11, 2006.
[10] W.-Y. Chen et al., "Wheelchair-Related Accidents: Relationship With Wheelchair-Using Behavior in Active Community Wheelchair Users," Archives of Physical Medicine and Rehabilitation, vol. 92, no. 6, pp. 892-898, 6// 2011, doi: http://dx.doi.org/10.1016/j.apmr.2011.01.008.
[11] B. Erickson et al., "The dynamics of electric powered wheelchair sideways tips and falls: experimental and computational analysis of impact forces and injury," Journal of neuroengineering and rehabilitation, vol. 13, no. 1, p. 20, 2016.
[12] B. E. Dicianno et al., "The Voice of the Consumer: A Survey of Veterans and Other Users of Assistive Technology," Military medicine, 2018.
[13] S. Arthanat, J. M. Desmarais, and P. Eikelberg, "Consumer perspectives on the usability and value of the iBOT® wheelchair: findings from a case series," Disability and Rehabilitation: Assistive Technology, vol. 7, no. 2, pp. 153-167, 2012, doi: doi:10.3109/17483107.2011.589487.
[14] "Viking 4x4." http://www.planetmobility.com/store/wheelchairs/power/viking/ (accessed.
[15] J. Candiotti, D. Kamaraj, R. Cooper, B. Daveler, and G. Grindle, "Usability Evaluation of a Curb-climbing Power Wheelchair for Indoor/Outdoor Accessibility," Archives of Physical Medicine and Rehabilitation, vol. 100, no. 10, p. e12, 2019.
[16] S. Sivakanthan et al., "Usability evaluation of attitude control for a robotic wheelchair for tip mitigation in outdoor environments," Medical Engineering & Physics, vol. 82, pp. 86-96, 2020.
[17] R. L. Kirby, J. Swuste, D. J. Dupuis, D. A. MacLeod, and R. Monroe, "The Wheelchair Skills Test: a pilot study of a new outcome measure," Archives of Physical Medicine and Rehabilitation, vol. 83, no. 1, pp. 10-18, 2002.
[18] J. L. Candiotti, A. Neti, S. Sivakanthan, and R. A. Cooper, "Analysis of Whole-Body Vibration Using Electric Powered Wheelchairs on Surface Transitions," Vibration, vol. 5, no. 1, pp. 98-109, 2022.