Edward M. Giesbrecht1
1University of Manitoba, Department of Occupational Therapy
INTRODUCTION
A manual wheelchair (MWC) can be effective in improving mobility for persons with disabilities. Mobility impairment is not uncommon, with 9.6% of Canadians identifying such a disability [1]. Among those with a mobility impairment, nearly 200,000 use a MWC to get around [2]. However, a comprehensive assessment and appropriate prescription that addresses the specific user's situation is essential if that MWC is to effectively address contextual barriers [3]. An important component of such an assessment is determining the method by which the user will propel their MWC, and then subsequently configuring the chair appropriately to support optimal and efficient propulsion. A majority of MWC users propel their chair using upper extremities; consequently, MWCs are commonly constructed and configured specifically for upper extremity propulsion. This configuration requires the seating surface to be raised high enough that the footrests sufficiently clear the ground and small obstacles. However, there are a substantial number of MWC users who propel their chair using lower extremities while seated in the chair (i.e., foot propulsion). Foot propulsion is more efficient and less effortful than traditional hand rims, so individuals with sufficient lower extremity function and strength (or those with inadequate upper extremity strength) may prefer this method [4]. For those who foot propel, the standard MWC configuration positions the seating surface far too high and makes it difficult to reach the ground. As a result, these individuals often slide forwards in their chair (in an attempt to gain better footing), which creates a number of issues: poor postural positioning is both uncomfortable and can create additional postural issues; there is a greater risk of sliding out of the chair and sustaining an injury; and the anatomical and biomechanical effectiveness of foot propulsion is compromised [5]. From a rehabilitation perspective, it is critical that therapists consider these issues and make appropriate, evidence-informed recommendations for MWC configuration specifically for those who foot propel.
Unfortunately, there is very limited evidence available to inform rehabilitation therapists' clinical reasoning regarding wheelchair configuration for those who foot propel. Wheelchair prescription guidelines suggest that reducing the seat height can address the issues identified above but provide little guidance about how much lower the seat height should be. Murata et al. [6] conducted a small study (n=7) and found significant graduated improvements in speed, stride length and lower extremity range of movement (ROM) as the seat was lowered from +60 mm to -60 mm from the popliteal fossa. However, outcomes deteriorated as the seat was lowered beyond -40 mm, suggesting that the seat eventually becomes too low and restricts the user's ability to perform an effective gait pattern. Heinrichs et al. conducted two studies of single foot propulsion simulating hemiplegic wheelchair users: one addressing forward propulsion [7] and the other backwards propulsion [8]. In both cases, there were significant improvements in propulsion speed, effectiveness, and perceived difficulty as the seat was lowered from +5cm to -5cm from the popliteal fossa. However, the difference in effect was smaller amongst the lowest heights. In addition, this study did not evaluate bi-lateral foot propulsion but rather considered the foot component of hemiplegic propulsion (i.e., using one hand and one foot).
Another potential option for MWC configuration is seat inclination. Some guidelines suggest that a slight forward slope (i.e., seat higher at the back and lower at the front) provides a more efficient posture and access for foot propulsion. Suzuki [9] is the only published study reporting on seat inclination and found a significant improvement in propulsion speed and reduction of muscle activity required in the hamstrings and trunk. However, this was a small study (n=10) with only a single condition (10° inclination) which used the single foot (hemiplegic) propulsion technique.
In summary, the existing evidence available to inform clinical practice regarding seat height and seat angle for individuals who bilaterally foot propel a MWC is extremely limited. Rehabilitation therapists might presume that a lower seat height is beneficial and that a forward seat slope may be helpful but have little guidance about how much lower or how much of a forward slope is optimal. This is particularly important given the limited evidence available also suggests that too much of an adjustment may prove to be equally restricting for the user. Obtaining more precise comparisons between configuration conditions with a larger sample and conducting the assessment with a bilateral foot propulsion technique will provide evidence that can be directly informative to clinical practice among rehabilitation therapists.
Purpose and Objectives
The purpose of this study is to explore the effect of differences in seat height and seat angle (inclination) on bilateral foot propulsion when using a MWC. The specific objectives are to determine whether differences in seat height and seat angle impact: a) propulsion speed; b) knee range of motion used during propulsion; c) effectiveness of foot propulsion gait; and d) perceived difficulty with foot propulsion.
METHODS
This study uses a within-subject repeated measures design. A repeated measures designs allows comparison between 5 different seat height conditions and 4 different seat angle conditions to determine whether there are significant differences in outcome, and if so, which conditions provide better outcomes. Participants propel a customized MWC through a standardized course using each seat configuration; the sequence is randomized to reduce any learning effects.
Participants
Study participants are students enrolled in the Occupational Therapy program at the University of Manitoba. We selected able-bodied individuals to provide a homogenous sample, to eliminate the need to accommodate for customized seating components within the test MWC, and to facilitate recruitment within a short time frame. For inclusion, students must be able to comfortably propel a MWC with both lower extremities; those with hip width exceeding 20 inches or lower leg length longer shorter than 15 inches are excluded as the test MWC will not accommodate these dimensions. All participants will provide informed consent. A power analysis was conducted using G*Power 3.1. Propulsion speed was selected as the primary outcome as it has consistently reported change, with a medium effect size being demonstrated by Heinrichs et al. [7,8]. For a repeated measures ANOVA with effect size of 0.25, alpha value of 0.05, and power value of 0.90, the required sample size is 26 participants.
Procedures
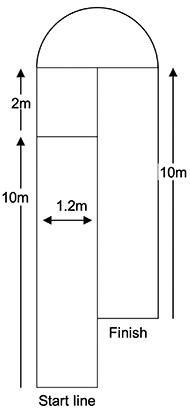
Measurement of thigh and lower leg length is conducted by one investigator using a standardized approach to ensure inter-rater reliability. The investigator applies an elastic strap around the thigh and lower leg and secures one XsensTM [10] sensors to each strap. To reduce risk of a practice effect and aborted data collection trials, participants are given instructions and have opportunity to practice bilateral foot propulsion using a standard MWC. Participants are then asked to sit in the testing MWC. The test MWC is an Everest & JenningsTM Traveler model with modifications. The folding frame has been reconfigured and welded to prevent flexing and an industrial jack mechanism integrated below the seat pan to allow rapid and precise changes to the seat height. In addition, the seat pan is hinged at the front and blocks constructed to support the back of the seat pan in precise angles of forward inclination. An articulating support arm is fastened to the frame of the wheelchair to mount a mobile phone for video capture. Seat depth is configured by inserting foam blocks between the participant and the backrest until there is 4-inch gap between the anterior edge of the seat and popliteal fossa. A 1" thick gel seat cushion demonstrates 5/8" immersion of the ischial tuberosities, raising seat height by 3/8".
The test course is ~ 28 m in total length. It begins with a 10-m straightaway followed by a 2-m stretch to prepare for a 180° turn around a pylon and finishing with another 10-m straightaway (see Figure 1). The course is 1.2 m wide. Lines are marked on the floor at the starting point, initial 10-m stretch, and the finish point. The test MWC is aligned with the casters in a trailing position, just touching the start line for each trial, and participants will have both feet on the floor and toes just touching the start line. Participants complete 8 trials of the test course. To assess seat height, there are 5 conditions (height adjustments to the MWC seat): lower leg (LL) length; LL + 1"; LL – 1"; LL – 2"; and LL – 3". The seat height is manually adjusted using a crank mechanism at the back of the wheelchair while the participant remains seated, and the height confirmed using a tape measure by a single CI. To assess seat angle, there will be 4 conditions: 0˚ (horizontal); 3˚; 6˚; and 9˚ of forward. While the seat angle is being adjusted, participants will be asked to stand up briefly during the adjustment and then sit back down in the test MWC. In each seat angle condition, the seat height is set at LL length; therefore, the LL height and 0˚ inclination condition is used for both the height comparison and the angle comparison (i.e., only 8 trials are required to capture the 9 test conditions). The order of conditions will be randomized in advance for each participant.
Before data collection, an investigator begins video recording on one mobile phone and initiates Xsens data collection on a second mobile phone. Upon completion of each trial, data collection is stopped and the data files (video .mov file and XSensTM .csv files) are airdropped to a laptop computer. An investigator then administers the Perceived Difficulty Questionnaire (PDQ) questionnaire.
Outcome measures
Objective 1: Propulsion speed will be determined from the video recordings, broken down into Initial 10m speed and Total Test Course speed. QuickTimeTM software is used to document the time the front casters touch the start, 10m, and course completion lines. Mean speed is calculated by dividing the two distances (10m and 28m) by the corresponding time to obtain an outcome in m/s.
Objective 2: Knee range of motion (ROM) is measured using XsensTM accelerometer/gyroscopic sensors and mobile app. The sensors record thigh and lower leg limb segment positions (relative to vertical), and knee joint angle is obtained from the difference between these positions (in degrees). The maximum and minimum values of knee flexion represent the ROM employed during propulsion, with a larger ROM providing a more efficient propulsion pattern.
Objective 3: Effectiveness of foot propulsion gait will be evaluated using the Wheelchair Propulsion Test (WPT) developed by Askari et al. [11]. The WPT is a simple, valid and reliable measure of propulsion for either upper or lower extremity propulsion techniques. Outcomes are established by calculating propulsion speed (distance in m/time in s = m/s); push frequency (# of push cycles/time in s = cycles/s); and finally push effectiveness (# of push cycles over 10m = m/cycle). These outcomes will also be obtained by viewing the video recordings.
Objective 4: Perceived difficulty with foot propulsion will be subjectively evaluated using the Perceived Difficulty Questionnaire (PDQ) [8]. The PDQ is a 5-point Likert scale, rating from "0" (very easy) to "4" (very difficulty). The PDQ has been used successfully in two previous lower extremity wheelchair propulsion studies [8,9].
Data Analysis
All analyses are conducted separately for a) 5 height conditions and b) 4 angle conditions. For all continuous data, a repeated measures ANOVA statistic is used to compare across conditions to determine if there is a statistically significant difference; if the omnibus test is significant, a Tukey's post-hoc analysis is conducted to determine differences between individual conditions. This will apply to mean Propulsion speed (with Initial 10m and Total Test Course time analyzed separately), mean Knee ROM, and Propulsion Effectiveness.
For the PDQ scale, which is ordinal data, Friedman's Test is used to compare across conditions to determine if there is a statistically significant difference; if the omnibus test is significant, a Wilcoxon test is conducted between each test pair to determine differences between individual conditions.
RESULTS
The study is currently conducting recruitment and data collection with participants. We anticipate that data collection will be complete by April 2023, at which time we will be able to report on the specific results and findings of the study.
DISCUSSION
Despite the substantial number of individuals who use bilateral foot propulsion for MWC mobility, there is surprisingly little evidence for rehabilitation practitioners and MWC prescribers to employ in their clinical reasoning for MWC configuration. While prescription guidelines and clinician experience suggest that a lower seat height enhances ease and (potentially) efficiency of propulsion, there is a point of diminishing returns where seat height is so low that it becomes counter-productive. Trial and error evaluation can be time-consuming, particularly when adapting low seat heights in MWCs designed for upper extremity propulsion; establishing evidence around optimal seat height could expedite this process. Furthermore, while some sources advocate for the benefits of forward seat inclination with bilateral foot propulsion, there is a paucity of evidence to confirm this recommendation, let alone provide guidance on the extent of seat slope that might have a desirable impact. We anticipate that the findings from this study will provide a more substantive base of evidence for clinicians to consider when configuring MWCs for bilateral foot propellers. Based on the findings, we also anticipate identifying direction for "next steps" in expanding evidence in this area of practice.
CONCLUSION
We anticipate findings from this study will be of significant value to clinicians who prescribe MWCs for individuals who use bilateral foot propulsion. In particular, we expect more granularity in the specific range of seat height that optimizes bilateral foot propulsion, including comparatively to unilateral foot propulsion (i.e., hemi-propulsion). We also expect to have preliminary evidence for benefits that might be incurred through forward seat inclination.
REFERENCES
[1] Morris, S. P., Fawcett, G., Brisebois, L., & Hughes, J. (2018). A demographic, employment and income profile of Canadians with disabilities aged 15 years and over, 2017. Statistics Canada: Catalogue no. 89-654-X2018002. https://www150.statcan.gc.ca/n1/en/pub/89-654-x/89-654-x2018002-eng.pdf?st=88PQHMdI
[2] Smith, E. M., Giesbrecht, E. M., Ben Mortenson, W., & Miller, W. C. (2016). Prevalence of wheelchair and scooter use among community-dwelling Canadians. Phys Ther, 96(8), 1135–1142.https://doi.org/10.2522/ptj.20150574
[3] Gowran RJ. (2012). Wheelchair and seating provision special issue. Irish J Occup Ther, 39(2).
[4] Charbonneau, R., Kirby, R. L., & Thompson, K. (2013). Manual wheelchair propulsion by people with hemiplegia: Within-participant comparisons of forward versus backward techniques. Arch Phys Med Rehabil, 94(9), 1707–1713.https://doi.org/10.1016/j.apmr.2013.03.001
[5] Buck, S. (2004) Wheelchair propulsion by foot: assessment considerations. Top Stroke Rehabil, 11:4, 68-71. doi: 10.1310/XNYL-VDEE-06QJTQ8E
[6] Murata, T., Asami, T., Matsuo, K., Kubo, A., & Okigawa, E. (2014). Effects of wheelchair seat-height settings on alternating lower limb propulsion with both legs. Assist Technol, 26(3), 151–156. https://doi.org/10.1080/10400435.2014.888108
[7] Heinrichs, N. D., Kirby, R. L., Smith, C., Russell, K. F. J., Theriault, C. J., & Doucette, S. P. (2021). Effect of seat height on manual wheelchair foot propulsion, a repeated-measures crossover study: part 1 – wheeling forward on a smooth level surface. Disabil Rehabil Assist Tech, 16(8), 831–839. https://doi.org/10.1080/17483107.2020.1741036
[8] Heinrichs, N. D., Kirby, R. L., Smith, C., Russell, K. F. J., Theriault, C. J., & Doucette, S. P. (2022). Effect of seat height on manual wheelchair foot propulsion, a repeated-measures crossover study: Part 2 - wheeling backward on a soft surface. Disabil Rehabil Assist Tech, 17(3), 325–330.https://doi.org/10.1080/17483107.2020.1782490
[9] Suzuki, T., Fukuda, J., & Fujita, D. (2012). Effects of forward tilt of the seat surface on trunk and lower limb muscle activity during one-leg wheelchair propulsion. J Phys Ther Sci, 24(3), 287–290.https://doi.org/10.1589/jpts.24.287
[10] Xsens dot wearable sensor platform. (n.d.) Xsens. Retrieved December 20, 2022, from https://www.xsens.com/xsens-dot
[11] Askari, S., Kirby, R. L., Parker, K., Thompson, K., & O'Neill, J. (2013). Wheelchair propulsion test: development and measurement properties of a new test for manual wheelchair users. Arch Phys Med Rehabil, 94(9), 1690–1698. https://doi.org/10.1016/j.apmr.2013.03.002