Junghan Sim, Patricia Karg, David Brienza
University of Pittsburgh, Department of Rehabilitation Science and Technology (Pittsburgh, PA USA)
INTRODUCTION
Pressure injuries are one of the most common medical challenges that negatively influence patient mortality, financial burdens for patients, their families and the health care system, and quality of life [1]. Wheelchair users are at a high risk for development of pressure injuries. Pressure injuries are a significant secondary complication for wheelchair users [2].
The purpose of a wheelchair cushion is to provide comfort and protection for wheelchair users by reducing pressure at support surfaces, a function that has been effective in decreasing the risk of pressure injury [3]. Wheelchair seat cushion designs are developed to reduce risk factors for pressure injuries. With advanced technologies, cushion manufacturers continue efforts to incorporate new strategies for pressure redistribution, shear and friction reduction, and dissipation of heat and moisture. Most of the technologies focus on reducing pressure, shear, and friction. In comparison, the reduction of heat and moisture seems to have been overlooked.
Microclimate between the user and the wheelchair cushion is represented by temperature and relative humidity [4]. Skin temperature increases beyond normal temperatures of skin exposed to ambient air cause increased perfusion. The response may be connected to a protective mechanism to avoid local overheating [5]. The result of increased skin temperature is accelerated heat transport to deeper tissue layers resulting in increased metabolism and higher susceptibility to the development and severity of pressure injuries [6]. Excessive moisture can damage skin due to maceration and associated loss of mechanical strength and increased risk of infection [7]. A high moisture environment results in a higher coefficient of friction between the skin and contacting textiles [8]. The greater the tendency the skin has to stick to the interface material, the greater the likelihood that there will be harmful deformation [9].
Our goal was to develop a prototype wheelchair cushion cover with microclimate management for pressure injury prevention. The objective of the design was to improve microclimate at the seat interface for wheelchair users by limiting heat and moisture accumulation. The second objective was to assure the wheelchair cushion cover was compatible with a broad range of currently available wheelchair cushions and did not negatively affect other beneficial wheelchair cushion performance characteristics.
DESIGN AND PROTOTYPING
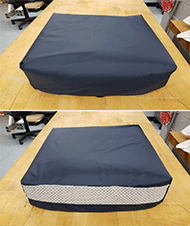
Product specifications were determined using a matrix which detailed the relationship between user needs and technical requirements. We determined needs and technical requirements based upon the literature, expert input and observations in our local seating and mobility clinic.
The product specifications were benchmarked against automobile seat and mattress industry technology. An iterative design method was completed. Results were narrowed to a single candidate solution for microclimate management at the seat interface (Figure 1).
The resulting prototype uses a modified Skin IQ™ (Arjo, Sweden) mattress cover for application on wheelchair seat cushions. The Skin IQ product provides a simple, effective solution to manage the skin microclimate of patients and thus help prevent pressure injuries. The system operates similar to a mattress with a traditional low air loss feature in that the outer layer of the cover is made from a vapor permeable material that allows water vapor to permeate through to the layers below. A second layer is a moisture and liquid permeable layer with a two-way stretch characteristic. The two-way stretch feature permits the cover to conform to the shape of the body-cushion interface without concentrating forces. Air flow is directed within the cushion underneath the vapor permeable layer by pulling air out to remove high humidity air and replacing it with drier air drawn in from the environment. Heat is also removed along with the moist air. Airflow is facilitated by the presence of a third layer, a spacer material located between the first and second layers, that serves to preserve airways in the cover even under compressive loading conditions. Unique features of the seat cushion cover prototype are that the outer layer was designed to completely envelop the top and sides of the cushion and allow the cover to conform to the shape of various wheelchair cushions, and the bottom part of the cover acted as a pocket for the wheelchair cushion in order to hold the position of the cover and prevent migration between the wheelchair cover and cushion. Although not implemented in the version evaluated for this paper, we propose to add an air-drying system using a desiccant filter combined with the spacer material or an air intake vent. Drying the air inside the cover or as it enters the cover allows humidity control even in high humidity environments where the system would otherwise have reduced ability to control moisture.
PROTOTYPE EVALUATION METHODS
Cushions Selected
Three cushion designs were selected for the benchtop laboratory evaluation (Table 1).
Cushion | Manufacturer | Cushion Design | HCPCS Code |
---|---|---|---|
J3 | Sunrise Medical | Viscous fluid and Contoured Elastic Foam | E2622 (Skin Protection, Adjustable) |
Quadtro Select High Profile | ROHO | Segmented Air Cell | E2624 (Skin Protection and Positioning, Adjustable) |
Vector | Comfort Company | Independent Air Cell | E2624 (Skin Protection and Positioning, Adjustable) |
* cushions tested were approximately 16x16 inch |
Evaluation Methods
The tests used to evaluate the cushions' performance were selected from the ISO16840 standards series.
ISO 16840-2:2018 - Loaded Contoured Depth and Overload Deflection
The loaded contour depth (LCD) test measures the immersion capability of a cushion or the ability to accommodate the pelvis [10]. A vertical load of 135N, 180N and 225N was applied to the indenter on the cushions. The vertical displacements of the indenter were recorded. The average of the three trials were used.
ISO 16840-6:2015 - Interface Pressure Measurement
Interface pressure measurement helps determine wheelchair cushion performance by measuring the magnitude and distribution of forces under simulated loading conditions [11]. Interface pressure was calculated by zones in five trials. The base point zones (BPZ) corresponding to the ischial tuberosities were defined. The BPZ was divided into the right base zone (RBZ) and the left base zone (LBZ). The rear center zone (CZ) make up the area behind BPZ and corresponds to the sacral-coccyx region. The Peak Pressure Index (PPI) in each BPZ were calculated by the greatest sum of pressures in a 9-10 area. Dispersion index was calculated from the sum of the pressure readings in RBZ, LBZ, and CZ divided by the sum of all pressure readings. The contact area was defined as the area of pressure readings, whose values were 5 mm Hg or greater.
ISO 16840-2:2018, Annex C - Horizontal Stiffness
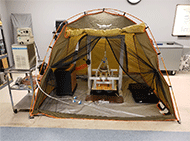
The horizontal stiffness test characterizes a cushion's ability to absorb horizontal perturbations at the interface between the cushion and the buttocks [10]. The rigid cushion loading indenter (RCLI) was applied onto the cushions. The MTS applied a relative horizontal displacement to the RCLI of 10 mm ± 1 mm and maintained the displacement for 60s ± 5 s. The peak and final horizontal force were recorded and the mean of the three trials used.
ISO 16840-7 Working Draft:2018 - Cushion Heat and Water Vapor Test
The test for heat and water vapor transmission properties of wheelchair cushions under simulated loading conditions is shown in Figure 2 [12]. The testing environment of 23±2°C and 50%±5% relative humidity (RH) was maintained with environmental controls using an external humidifier in the tent. The water circulator was also maintained between 35°C and 37°C, and 200 ml of distilled water was added in a thermodynamic rigid cushion loading indenter (TRCLI). The data recording began with temperature and humidity readings from the sensors at a 0.2 Hz sample rate. At steady state, the TRCLI was applied onto the cushion. After 180±6 minutes, the TRCLI was lifted above the cushion. The indenter was then returned to the same position on the cushion and the data was recorded by the sensors for 15 minutes. The data recorded included the temperature and humidity at the beginning of the test (T0), 60 minutes (T60), 120 minutes (120), 180 minutes (T180), at the pressure relief lift (T181), and at 196 minutes (T196). The heat and water vapor data were retrieved at 6 points throughout each 3 hours trial. The average of the three trials was used for each cushion with and without the prototype cover.
RESULTS
The table below and Figure 3 present the comparison of results for the cushions with and without the prototype cover. The analyses were conducted on IBM SPSS Statistics 25 software (Chicago, IL). A Shapiro-Wilk test was chosen for the normality test. A paired t-test was used for the normally distributed data, while a Wilcoxon signed rank test was performed for non-parametric distributions. Due to the multiple comparisons, a Bonferroni correction was used. An adjusted p-value of 0.002 was regarded as a significant difference.
Method | Variable | Cushions with Prototype | Cushions without Prototype | Sig. (2-tailed) | ||
---|---|---|---|---|---|---|
Mean | SD | Mean | SD | |||
LCD Test | LCD (mm) | 70.92 | 3.21 | 66.20 | 3.16 | 0.008 |
L180 (mm) | 4.78 | 0.55 | 3.56 | 0.44 | 0.014 | |
L225 (mm) | 9.56 | 0.78 | 7.56 | 0.69 | 0.128 | |
Interface Pressure Measurement | PPI in RBZ (mmHg) | 58.06 | 5.84 | 66.11 | 6.38 | 0.001 |
PPI in LBZ (mmHg) | 55.76 | 4.20 | 65.85 | 2.70 | 0.000 | |
Dispersion Index (%) | 36.65 | 4.42 | 38.13 | 3.44 | 0.242 | |
Contact Area (![]() |
100460 | 4185 | 97933 | 5185 | 0.101 | |
Horizontal Stiffness | Peak Horizontal Force (N) | 82.46 | 6.94 | 114.89 | 11.91 | 0.009 |
Final Horizontal Force (N) | 59.69 | 4.96 | 88.85 | 8.84 | 0.010 | |
Cushion Heat and Water Vapor Test | T0 (% RH) | 48.45 | 0.62 | 48.39 | 1.27 | 0.957 |
T60 (% RH) | 49.64 | 0.49 | 71.18 | 1.10 | 0.000 | |
T120 (% RH) | 47.90 | 0.83 | 76.16 | 1.06 | 0.000 | |
T180 (% RH) | 47.63 | 0.74 | 78.48 | 0.92 | 0.000 | |
T181 (% RH) | 43.78 | 0.67 | 69.94 | 1.36 | 0.000 | |
T196 (% RH) | 47.24 | 0.84 | 74.95 | 1.40 | 0.000 | |
T0 (℃) | 25.94 | 0.55 | 25.52 | 0.60 | 0.411 | |
T60 (℃) | 31.97 | 0.22 | 32.08 | 0.30 | 0.675 | |
T120 (℃) | 32.65 | 0.07 | 33.26 | 0.19 | 0.016 | |
T180 (℃) | 32.68 | 0.10 | 33.40 | 0.24 | 0.017 | |
T181 (℃) | 30.89 | 0.36 | 31.71 | 0.21 | 0.022 | |
T196 (℃) | 32.49 | 0.12 | 33.19 | 0.27 | 0.047 |
DISCUSSION
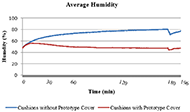
Given the heat and water vapor test results, the humidity level of cushions with the prototype cover was lower than that of the cushions without the cover. The humidity was maintained at approximately 50% humidity and temperature was found to be lower at all time points (T60, T120, T180, T181, and T196) except at the beginning of the test (T0). The optimal microclimate for pressure injury prevention is currently unknown [8]. However, stratum corneum (SC) hydration plays a significant role in tissue properties. The stable status of the SC ranges from 40% to 60% RH, and SC hydration is accelerated over 60% RH [13]. Importantly, moisture increases the coefficient of friction between skin and textiles thus increasing the likelihood that shear forces will result in harmful tissue deformation. Therefore, maintaining a humidity level around 50% is a logical target for pressure injury prevention. The resulting maintenance of 50% RH was likely linked to the ambient RH controlled at 50%±5%.
The mechanical characterization testing indicated the prototype cover did not negatively impact load-bearing performance. A statistically significant decrease in PPI in RBZ and LBZ was observed between the cushions with and without the prototype cover. More specifically, the cushion with the prototype cover provided additional pressure distribution. Our result is consistent with Xiaohua, et. al. who demonstrated lower pressure for a polyurethane foam mattress with spacer fabric compared to the same mattress without spacer fabric [14].
CONCLUSION
We demonstrated that the wheelchair cushion cover with microclimate management effectively controls moisture at its top surface in a range near 50% RH. The results of standard performance tests for wheelchair cushions with the prototype indicated that the cover provided additional pressure distribution and did not negatively influence select other mechanical load-bearing characteristics.
REFERENCES
- Bauer, K., Nazzal, M., Jones, O., & Qu, W. (2016). Pressure Ulcers in the United States' Inpatient Population From 2008 to 2012: Results of a Retrospective Nationwide Study. Ostomy Wound Management, 28(3), 70–77.
- Regan M, Teasell RW, Keast D, Aubut JL, Foulon BL, Mehta S. (2010). Pressure Ulcers Following Spinal Cord Injury.
- Brienza, D., Kelsey, S., Karg, P., Allegretti, A., Olson, M., Schmeler, M., … Holm, M. (2010). A Randomized Clinical Trial on Preventing Pressure Ulcers with Wheelchair Seat Cushions. Journal of the American Geriatrics Society, 58(12), 2308–2314.
- NPUAP (2014). Prevention and Treatment of Pressure Ulcers: Clinical Practice Guideline.
- Petrofsky, J. S., Berk, L., Alshammari, F., Lee, H., Hamdan, A., Yim, J. E., Al-Nakhli, H. (2012). The Interrelationship between Air Temperature and Humidity as Applied Locally to the Skin: The Resultant Response on Skin Temperature and Blood Flow with Age Differences. Med. Sci. Monit., 18(CR201–208).
- Kokate, J., Leland, K. J., Held, A. M., Hansen, G. L., Kveen, G. L., Johnson, B. A., … Laizzo, P. A. (1995). Temperature-modulated Pressure Ulcers: A Porcine Model. Arch. Phys. Med. Rehabil., 76, 666–673.
- Nakgami, G., Sanada, H., Kitagawa, A., Tadaka, E., Maekawa, T., Nagase, T., & Konya, C. (2006). Incontinence Induces Stratum Corneum Vulnerability and Impairs the Skin Barrier Function in the Perianal Region. Dermatology, 213, 293–299.
- Persson, B. N. J., Kovalev, A., & Gorb, S. N. (2013). Contact Mechanics and Friction on Dry and Wet Human Skin. Tribol. Lett., 50, 17–30.
- Sopher, R., & Gefen, A. (2011). Effects of Skin Wrinkles, Age and Wetness on Mechanical Loads in the Stratum Corneum as Related to Skin Lesions. Med. Biol. Eng. Comput., 49, 97–105.
- International Organization for Standardization. (2018). Wheelchair Seating - Part 2: Determination of Physical and Mechanical Characteristics of Seat Cushions Intended to Manage Tissue Integrity. ISO 16840-2:2018(E), Geneva, Switzerland.
- International Organization for Standardization. (2015). Wheelchair Seating - Part 6: Simulated Use and Determination of the Changes in Properties of Seat Cushions. ISO16840-6:2015, Geneva, Switzerland
- International Organization for Standardization. (2018). Wheelchair Seating - Part 7: Cushion Heat & Water Vapour Testing. ISO 16840-7:2018, Geneva, Switzerland.
- Imhof, R. E., De Jesus, M. E., Xiao, P., Ciortea, L. I., & Berg, E. P. (2009). Closed-chamber Transepidermal Water Loss Measurement: Microclimate, Calibration and Performance. Int. J. Cosmet. Sci., 31(97–118).
- Xiaohua, Y., Hong, H., & Xunwei, F. (2008). Development of the Warp Knitted Spacer Fabrics for Cushion Applications. Journal of Industrial Textiles, 37(3), 213–223. https://doi.org/10.1177/1528083707081592